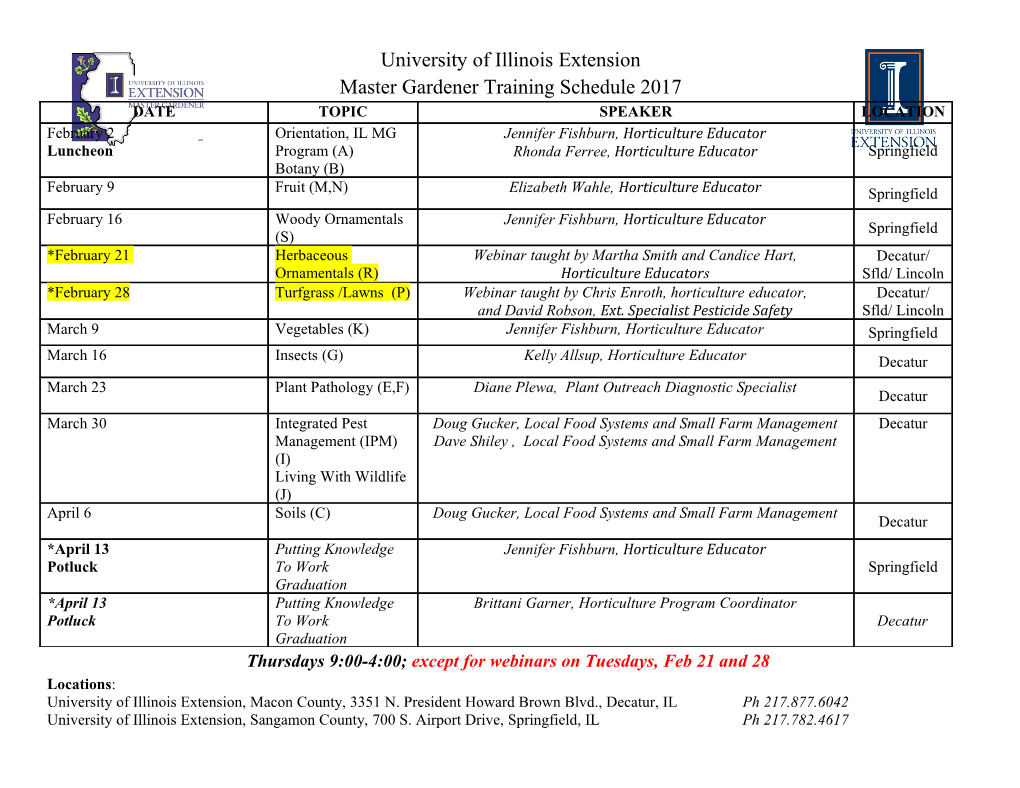
Abstracts 20 GeoBremen 17 The System Earth and its Materials – from Seafloor to Summit Joint Meeting of DGGV and DMG Bremen, Germany September 24 – 29, 2017 www.GeoBremen17.de Plenary Speaker Plenary Speaker A-696 Drilling the Chicxulub impact crater to understand planetary evolution and mass extinction Gulick, S.1, 2, Morgan, J.3, Expedition 364 Science Party 1Institute for Geophysics, University of Texas at Austin, Austin, Texas, United States, 2Deparment of Geological Sciences, University of Texas at Austin, Austin, Texas, United States, 3Imperal College, London, United Kingdom Content The most recent of Earth’s five largest mass extinction events occurred 66 Ma, coeval with the impact of a ~14 km asteroid into what is today the Yucatán Peninsula, México, producing the ~200 km-wide Chicxulub crater. This impact, by some estimations, drove the extinction of 75% of life on Earth at the genus level. The mass extinction event marks the boundary between the Cretaceous and Paleogene. Proposed kill mechanisms include thermal effects caused by the reentry of fast ejecta into Earth’s atmosphere, dust and sulfate aerosols reducing Earth’s solar insolation, ocean acidification, and metal toxicity due to the chemical make-up of the impactor. The magnitude and duration of these processes is still debated, and further evaluation of the proposed kill mechanisms requires an understanding of the mechanics of the Chicxulub impact as well as the resulting global environmental perturbations. In April and May 2016, the International Ocean Discovery Program, with co-funding from the International Continental Scientific Drilling Program, successfully cored into the Chicxulub impact crater with nearly 100% recovery. These cores include the first-ever samples of the transition from an intact peak ring through post- impact sediments. A peak ring is a discontinuous ring of mountains observed within the central basin of all large impact craters on rocky planets. Newly drilled cores include the uplifted target rocks, melt-rich impactites, hydrothermal deposits, a possible settling layer, and the resumption of carbonate sedimentation. The discovery that Chicxulub’s peak ring consists of largely granitic crust uplifted by ~10 km calibrates impact models, allows for observation of impact processes, and for a first-order assessment of the environmental consequences of the impact (“kill mechanisms”). These cores provide an unprecedented opportunity to study the relationship between the kill mechanisms, the mass extinction event, and the initial recovery of life. Plenary Speaker A-903 Interplay Between Magmatic, Tectonic and Hydrothermal Processes at Oceanic Spreading Centers Humphris, S.E. Geology & Geophysics Department, Woods Hole Oceanographic Institution, Woods Hole, MA, United States Content Significant progress has been made in recent years in understanding the interplay among the geological processes that produce the characteristics of oceanic spreading centers with different spreading rates. Along fast-spreading ridges, extension is mostly accommodated by magmatism. Magma supply is high resulting in frequent volcanic eruptions and relatively smooth seafloor topography. In contrast, at intermediate- and slow- spreading ridges, faulting with only intermittent volcanic activity accommodates a significant portion of the extension. In fact, the low magma supply at slow and ultraslow spreading ridges results in crustal accretion that is characterized by asymmetric exhumation along large-offset normal (detachment) faults and the formation of oceanic core complexes that expose lower crust and upper mantle rocks. Hydrothermal circulation at oceanic spreading centers is driven by heat from crystallization of magma and cooling of young oceanic lithosphere. At fast-spreading ridges, axial magma chambers at depths of 1-2 km result in relatively shallow depths of hydrothermal fluid circulation, but as spreading rate decreases, fluids focused along large faults can reach depths of perhaps 5 to more than 8 km. The role of faulting is most extreme at ultraslow-spreading ridges where permeability becomes increasingly important as a secondary control on hydrothermal vent field frequency. Despite the large variations observed in the chemical compositions of vent fluids discharging at mid-ocean ridges and at back-arc spreading centers, there are similarities in the fundamental processes — fluid-mineral equilibria, phase separation, magma degassing, interactions with overlying sediments — that contribute to vent fluid chemistry. Seawater entrained in deep-sea hydrothermal systems may chemically react with various rock types, ranging from mafic (basalt, gabbro) and ultramafic rocks (peridotite) at mid-ocean ridges to more felsic volcanic rocks (andesite, dacite, rhyolite) at back-arc spreading centers due to proximity to the subducting slab. Phase separation can occur at depth within the basement, resulting in the partitioning of chemical species and volatiles into different fluid phases. In some cases, particularly in back-arc settings, magmatic degassing that can add volatiles and metals to the circulating fluids. This complex interplay between magmatic, tectonic and hydrothermal processes facilitates the cycling of heat and mass between the Earth and the oceans. Plenary Speaker A-899 New discoveries on the Origin of Life: Darwin’s Warm Little Pond revisited Van Kranendonk, M. School of Biological, Earth and Environmental Sciences, The University of New South Wales Sydney, Kensington, NSW, Australia Content Darwin first postulated, 145 years ago, that life might have originated in “…some warm little pond with all sorts of ammonia and phosphoric salts, light, heat, electricity, etc. present, that a protein compound was chemically formed, ready to undergo still more complex changes…”. However, the 1970’s discovery of black smokers and their rich vent fauna, together with evidence from the mitochondrial tree of life, led to the currently prevailing view that life got started at deep sea vents. But it turns out that the energy required for polymerisation of organic molecules from simple amino acids comes from expelling a water molecule. In addition, the cytoplasm of cells from all three branches of life have K+/Na+ ratios more aligned with fresh water than seawater, and that certain key elements (B, Zn, Mn) required as catalysts for polymerisation are hard to concentrate in a marine environment. Thus, the oceans, or any permanently wet environment, is not suitable for prebiotic chemistry and a land-based environment undergoing wet-dry cycles is required for an Origin of Life setting. What kind of environment? Previous authors have suggested a desert with occasional rain, or a river flowing over komatiitic crust as possible sites for the Origin of Life. But these cold-water environments lack the capacity for compositional complexity and highly reactive interfaces. Recent discoveries from an ancient analogue site in Western Australia’s Pilbara region provide support for an Origin of Life in terrestrial hot springs. The 3.48 Ga Dresser Formation is well known as hosting some of Earth’s oldest convincing evidence of life (stromatolites, fractionated stable isotopes, putative microbial remains), all within an active volcanic caldera characterised by voluminous hydrothermal fluid circulation. Previously, the depositional environment was considered as shallow marine, but the recent discovery1 of geyserite and other siliceous sinter deposits containing biosignatures and spatially associated with stromatolites, point to a thriving terrestrial hot spring setting with wet-dry cycles. A parallel, key discovery is of concentrations of the key prebiotic elements (B, Zn, and Mn), arising from hot water-rock interactions. These discoveries are leading to a developing model for the Origin of Life in terrestrial hot spring fields, and have implications for Astrobiology and the search for life elsewhere in the Solar System. 1 Djokic, T. et al. (2017). Nature Communications 8:15263 Plenary Speaker A-910 Nonclassical nucleation and crystallization Cölfen, H. Physical Chemistry, University of Konstanz, Konstanz, Germany Content In recent years, research on nucleation and crystallization has revealed alternative pathways to the classical ones known from the textbooks of crystallization. These so called nonclassical pathways allow for a better understanding of so far difficult to understand crystallization processes as observed for example in Biomineralization. In addition, they open up entirely new possibilities for the control of crystallization processes and therefore crystals, which would be impossible to synthesize by classical methods. The presentation will start with nonclassical nucleation processes for the example of CaCO3. It can be shown that so called prenucleation clusters of ca. 40 ion pairs in size exist in equilibrium with the ions and ion pairs. These clusters are highly dynamic polymers which constantly form and dissociate. Their formation is entropically driven by the release of hydration water. Increasing the overall ion concentration leads to a decrease in their dynamics and a phase transition in form of binodal demixing to nanodroplets. These coalesce and form larger droplets which solidify and form amorphous CaCO3, which finally crystallizes. This multi-step nucleation scenario is not only relevant for CaCO3, but also other minerals like calcium oxalates or phosphates. Once nanocrystals are formed, they are expected to grow layer by layer according to the textbook
Details
-
File Typepdf
-
Upload Time-
-
Content LanguagesEnglish
-
Upload UserAnonymous/Not logged-in
-
File Pages702 Page
-
File Size-