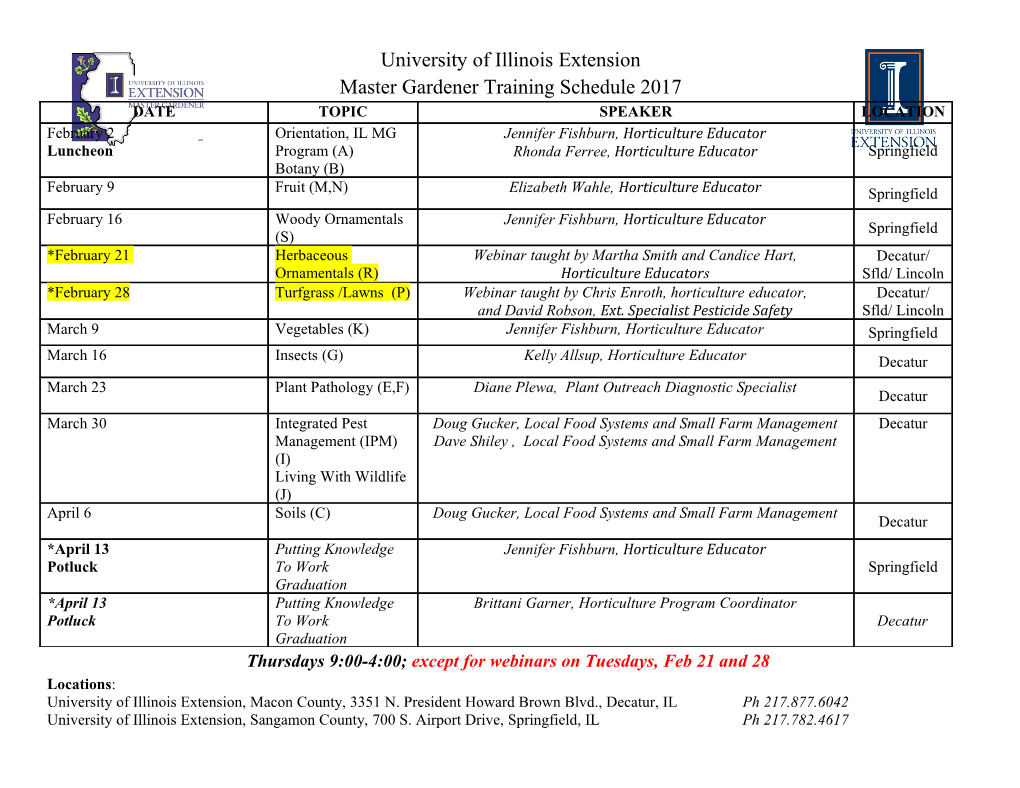
Chapter 12 APPLICATIONS OF LANDSCAPE GENETICS TO CONNECTIVITY RESEARCH IN TERRESTRIAL ANIMALS 1 2 3 Lisette P. Waits, Samuel A. Cushman, and Steve F. Spear 1 Fish and Wildlife Sciences, University of Idaho, USA 2 Forest and Woodlands Ecosystems Program, Rocky Mountain Research Station, United States Forest Service, USA 3 The Orianne Society, USA 12.1 INTRODUCTION heterogeneous landscapes. Finally, we end the chapter with future directions and current knowledge gaps for This chapter focuses on applications of landscape terrestrial animal landscape genetic studies. genetics for understanding connectivity of terrestrial animal populations. We start with a general introduc­ tion covering unique characteristics and challenges of 12.2 GENERAL OVERVIEW the terrestrial study system, followed by an overview of OF TERRESTRIAL ANIMAL STUDY common research questions addressed in terrestrial SYSTEMS AND RESEARCH animals using neutral markers in landscape genetics. CHALLENGES These common research questions include detecting and defining barriers, identifying corridors, examining Landscape genetic studies have focused on terrestrial source-sink dynamics, and detecting and predicting animals more than any other taxonomic group (Storfer animal responses to environmental change. Based on et al. 2010). This is not surprising given the inclusion of this overview, we highlight limitations of current “land” in the name of the discipline and the usefulness research approaches and present case studies that of landscape genetics as a method to infer animal have specifically dealt with some of these limitations movement, a key process in ecology and conservation. when testing ecological hypotheses about gene flow in Many aspects of landscape genetic studies are well Landscape Genetics: Concepts, Methods, Applications, First Edition. Edited by Niko Balkenhol, Samuel A. Cushman, Andrew T. Storfer, and Lisette P. Waits. © 2016 John Wiley & Sons, Ltd. Published 2016 by John Wiley & Sons, Ltd. 200 Applications of landscape genetics to connectivity research in terrestrial animals suited for applications related to terrestrial animals. dynamics also are very different between typical terrestrial Landscape genetics usually requires a model of the and aquatic systems. Functional connectivity in terrestrial continuous environment, and such GIS models are environments is often more constrained, occurs at a finer most widely available for terrestrial systems (Chap­ scale, and changes at a slower rate than connectivity ter 2). This has allowed for a more realistic representa­ across marine environments (Carr et al. 2003; also see tion of the heterogeneity present in terrestrial Chapter 13). In many ways, this can make it easier to landscapes, whereas it is much more difficult to repre­ develop strong statistical models in terrestrial landscape sent the heterogeneity in aquatic systems using typical genetic studies if sufficient spatial data are available, as remote sensing methods. The ability to incorporate the scale of sampling is more likely to match the scale of existing GIS data into studies of animal population the gene flow process. In contrast, studies of seascape connectivity has benefited researchers greatly. In addi­ genetics often have detected very little genetic structure tion, landscape genetics has enabled effective research across broad areas (Selkoe et al. 2010; Schunter et al. on animals that are secretive or difficult to study using 2011), due in part to the combination of long-distance traditional mark-recapture or radio telemetry methods transport of gametes and larva by currents and temporal (e.g., Schwartz et al. 2009). Furthermore, landscape dynamics that are rapid relative to population turnover. In genetic studies provide some of the best case studies of the terrestrial system, the dynamics of landscape change how contemporary habitat alteration is affecting pop­ are generally slower than population processes, enabling ulation processes such as connectivity in terrestrial the effects of landscape change to be recorded in changes species, ranging from insects (e.g., Keyghobadi et al. in the genetic structure of populations. However, in many 2005a), amphibians (e.g., Funk et al. 2005; Murphy instances rates of change in terrestrial landscapes exceeds et al. 2010a, 2010b; Spear et al. 2012), reptiles (e.g., the equilibration time of population genetic processes, Stow et al. 2001; Clark et al. 2010), birds (e.g., Pav­ requiring non-equilibrium methods to analyze current lacky et al. 2009), and mammals (e.g., Epps et al. 2005; rates of gene flow. Vignieri et al. 2005; Wasserman et al. 2012). The vast scales, high-frequency dynamics, and typi­ cally immense numbers of gametes and larva in marine systems create processes that are essentially “large 12.2.1 Comparison of terrestrial animal number problems” in the sense of statistical mechanics studies with other systems and the Boltzmann equation (Boltzmann 1872), in which the overall statistical behavior of the entire system Landscape genetics research focused on terrestrial ani­ is highly predictable as the outcome of millions of micro- mals has a number of unique aspects compared to scale interactions. Terrestrial systems, however, are aquatic systems or terrestrial plant species. The terres­ more often in the range of “middle number problems”, trial landscape can be depicted as a combination of linear where there are often too many interacting individuals elements, mosaics of categorical patch types (Forman and entities to effectively model the mechanistic inter­ 1995), and gradients of continuously varying ecological actions of the entire system, but too few to average away factors (McGarigal and Cushman 2005; Chapter 2). The the individual interactions in a statistical mechanics patterns of these factors interact with organism behavior approach, and dynamics are too slow to collapse them to affect population processes, such as mating and as “noise” and too frequent to ignore and model as a dispersal. The functional connectivity of a landscape static system. As a result, terrestrial landscape genetic depends on how the pattern of the environment interacts analysis is often a “complex systems” problem, in which with behavioral responses and movement abilities of the researchers attempt to reduce complexity by adopting species to drive population processes. spatially-explicit approaches to represent the location, Terrestrial systems are generally depicted as two-dimen­ movement, and interactions of individuals within popu­ sional patterns, which is in contrast to many marine and lations as functions of the spatial structure of the land­ lacustrine systems, in which hydrodynamic fluxes occur scape. This presents a number of conceptual and in three dimensions, and riverine systems, which are often technical challenges for analysis. For instance, a depicted as networks of one-dimensional links. This in researcher using resistance modeling (see Chapter 8) principle can make analysis of terrestrial landscapes sim­ typically has to parameterize a multivariate resistance pler than marine systems and more complicated than surface in a terrestrial system, whereas following ocean­ riverine networks, simply as a function of the dimension­ ographic currents or stream lengths may be sufficient in ality of the pattern–process relationships. Temporal an aquatic system (although many aquatic systems do General overview of terrestrial animal study systems and research challenges 201 have multiple environmental influences; Chapter 13). often more strongly affected by land conversion, roads, Finally, the slower rate of change in functional connec­ and other forms of habitat fragmentation (e.g., Stow et al. tivity in the terrestrial environment introduces the chal­ 2001; Proctor et al. 2005; Amos et al. 2014; Paquette lenge of accounting for temporal lags. et al. 2014; Elliot et al. 2014a, 2014b). Animals and plants also differ with respect to landscape The complex, often indirect, relationship of animal influence on genetic structure in several ways (also see movement with gene flow is another challenge (Boho­ Chapter 11). The most obvious difference is that individ­ nak 1999). Animal movement occurs for multiple rea­ ual plants are stationary whereas animals are mobile. sons, many of which are not related to gene flow. Thus, The second major difference is that gene flow in terres­ models used to account for landscape influence in a trial animals occurs through the transport of gametes genetic study must recognize that only a subset of by the individual, whereas movement of gametes in movements are relevant when using empirical move­ plants typically occurs independent of the individual ment data to identify key landscape variables (see plant through seed and pollen. Therefore, modeling plant Chapter 8). Furthermore, genetic data may not always gene flow might require a simultaneously modeling of be appropriate to direct conservation actions such as both gametes. On the other hand, the field of adaptive movement corridors if the types of movement the actions landscape genetics is much more amenable to plants due need to facilitate are not tied to mating or reproduction to their sessile nature (see Chapter 9). (i.e. migration). These concerns highlight the impor­ tance of understanding the study system and conducting landscape genetic studies with well-designed objectives 12.2.2 Methodological and study that can investigate multiple processes. design challenges often faced by When analyses are based on individuals, another
Details
-
File Typepdf
-
Upload Time-
-
Content LanguagesEnglish
-
Upload UserAnonymous/Not logged-in
-
File Pages21 Page
-
File Size-