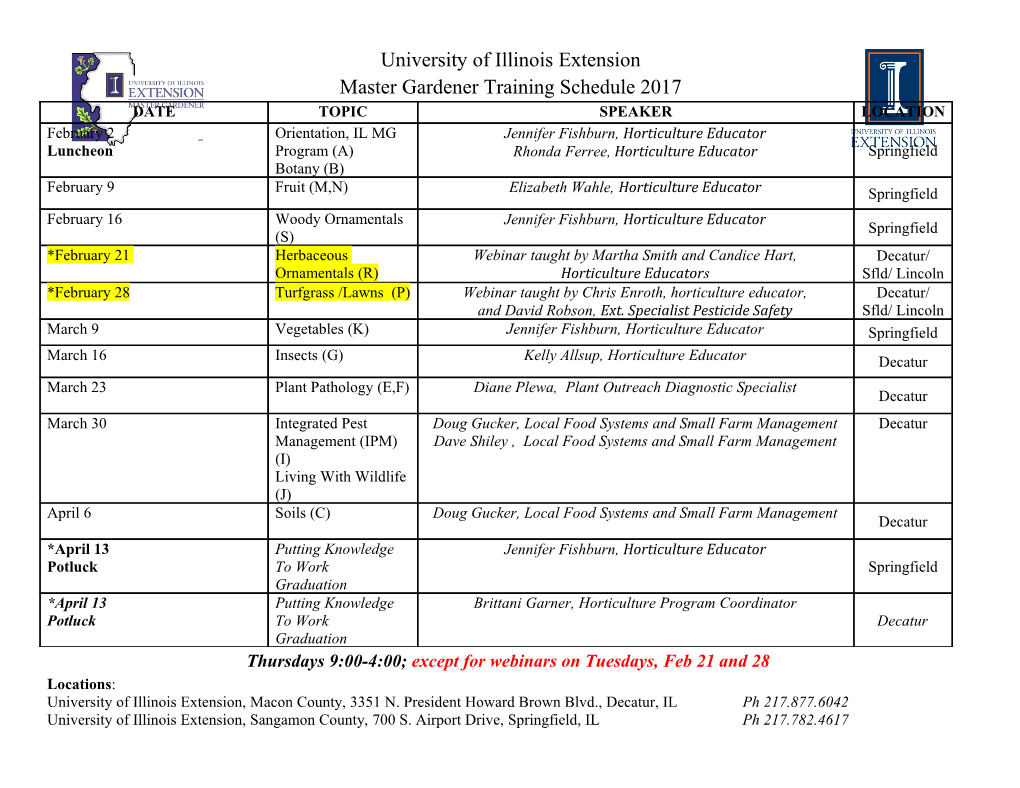
The role of vorticity in developing lineation by wind erosion MARION I. WHITNEY Central Michigan University, Mount Pleasant, Michigan 48859 ABSTRACT This paper reports some of the results of a 15-yr investigation, the central objective of which has been to determine the precise de- Experiments and field observations indicate that both the gross tails of how wind erosion forms lineations. I considered earlier that shaping and the sculpturing of lineation and pit details on wind- wind might produce lineations by shifting sand along linear trends eroded surfaces may be accomplished by the impact of particles until grooves were worn and that it might erode pits by continu- which have been impelled aerodynamically by interfacial flow or by ously cycling sand. Both hypotheses were quickly found to be faulty vorticity. The vorticity operates along lines of positive, negative, when put to test. Particles did not travel in contact with groove and secondary flow over all surfaces of an object. floors or cycle in pits. Also, escape from both types of features was The axis of vorticity is typically normal to or at some high angle too rapid to determine the escape patterns. In the laboratory, with to the surface undergoing erosion. Therefore, it is not the so-called oriented specimens and very low velocity, the escape problem was roller vortex type which has been postulated by other investigators, resolved (Fig. 1). The lineation problem, however, required actual for one typical erosion pattern left abundantly by the vortex observation of the erosion processes and extensive experimenta- configuration is a round pit either helically or radically scored. tion. Often such pits occur in chains along the beds of the lineations. A Most previous investigators have not utilized the data of second type of erosion pattern common in channels is parallel aerodynamics to explain wind erosion. Higgins (1956) postulated transverse lineation, which this writer has seen in development in aerodynamic erosion by means of suspended particles in interfacial snow flutes under influence of normal-axis vortices. Such vortices currents, those currents at the boundary of rock and air. Wilson travel singly along lines of flow, pulling particles centripetally into (1972) suggested that interfacial currents might influence deposi- the vortex configurations. In snow flutes, for example, vortices be- tion and referred to such currents as a poorly understood sub- come visible due to suspended snow, and the secondary flow can be sidiary flow system. I believe, after nearly 15 years of testing flow delineated. Where general windflow is essentially unidirectional, patterns on hundreds of ventifacts in a wide range of shapes, after the erosional result of such vorticity is the creation of cross- extensive study of vorticity, and after conducting nearly 12 years of lineated, essentially symmetrical grooves. wind-blast studies and extensive analyses of electron micrographs of surfaces prior to and following wind-blasting, that I now have a INTRODUCTION good knowledge of these interfacial flow systems. In addition, the experimental data are corroborated by extensive field data on ven- This study is a continuation of that reported by Whitney and tifacts, sand ripples, sand flutes, and snow erosion. Dietrich (1973). In that report, it was shown that (1) aerodynamic Aerodynamic erosion may involve several mechanisms, such as processes, including negative as well as positive flow and vorticity interfacial flow, abrasion, vorticity, lift, wind cleavage, and possi- (cyclic activity with axis essentially normal to the surface on which it operates), may act simultaneously on all surfaces to shape, UI sculpture, and burnish ventifacts; and (2) suspended dust-sized particles moving along interfacial flow lines may act as the erosive tools. Subsequently, Dietrich (1977) illustrated dust erosion of nonsoluble minerals with hardnesses of up to 6 (Figs. 3A, 3B, and 3C). The original plan was to divide this work into seven papers, some of which were to be field studies and others laboratory studies. When the first four of these papers were submitted, the editor re- quested that they be combined into one paper. While there are ob- vious advantages to the reader in such a compilation, much of the experimental and supporting data had to be removed from the ac- WIND DIRECTION count. To the readers who feel the lack of these types of data, I can only say that the work could not be at once consolidated and carry Figure 1. Diagram of a simple interfacial flow relationship over a sharp amply detailed proofs. Such proofs were sought, however, for every crest. "W" refers to wind direction, "p" to positive flow, and "n" to nega- concept from some combination of the following: field observa- tive flow. This basic plan can apply either to a rock as a whole, or snow or a tions, comparative studies of thousands of ventifacts, microscopy sand dune, or to a hill or to minor irregularities on larger features. The line of wind-eroded surfaces, flow and vorncity tests, erosion of spec- of negative flow is in contact with the surface at the foot of the slope where the pressure is high and velocity is low but pulls outward to meet the over- imens by wind-blasting, and electron microscopy of the test spec- riding positive flow where velocity is higher and pressure is lower. Vorticity imens. occurs at the juncture of the flow lines at the crest. Geological Society of America Bulletin, v. 89, p. 1-18, 9 figs., January 1978, Doc. no. 80101. 1 Downloaded from http://pubs.geoscienceworld.org/gsa/gsabulletin/article-pdf/89/1/1/3444012/i0016-7606-89-1-1.pdf by guest on 27 September 2021 2 M. I. WHITNEY bA Jtowtronie bly electrostatic-electromagnetic phenomena. All of these have perimentation have been flow-testing and wind-blasting. Flow- been investigated by me except the last. Helene Brewer, who testing was done on more than 1,000 oriented ventifacts where the suggested wind-blasting and who built the wind tunnels used and impact zones could be determined. This is possible on Michigan monitored the first wind-blast tests, is currently experimenting with dolomite ventifacts with rilled margins because impact areas are the electrostatic-electromagnetic aspect. The main modes of ex- bald, that is, devoid or nearly devoid of rills (Fig. 2A). On other Downloaded from http://pubs.geoscienceworld.org/gsa/gsabulletin/article-pdf/89/1/1/3444012/i0016-7606-89-1-1.pdf by guest on 27 September 2021 ROLE OF VORTICITY IN DEVELOPING LINEATION BY WIND EROSION 3 Figure 2. Ventifact forms, lineation, and pit features. Shaftless arrows indicate north orientation; "h" and "p" refer to high velocity and prevailing winds, respectively. Negative flow is indicated by an "n" beside an arrow. A. Top of dolomite ventifact with two principal rill sets or grooves, Big Sable Point, Michigan. B. Top of dolomite ventifact in which crestal and basal vortex pits met to form a perforation, Big Sable Point, Michigan. C. Top of dolomite ventifact with a large radially lineated vortex pit, Big Sable Point, Michigan. D. Top of keeled basalt ventifact, Shoshoni, Wyoming. E. Lift of the white grain from the pit occurs at extremely low velocity on negative flow. F. Lineated basalt boulder ventifact, Sleeping Bear moraine, Michigan. Approx- imately 1 m long. G. Profile of polished Pleistocene ventifact, showing undercut facet and basal concavity, gravel pit near Muir, Michigan (0.75x). H. Graywacke ventifact used in the experiment in Figure 2E, Big Sable Point, Michigan (0.75 x). I. Lateral view of chalcedony ventifact showing a number of wedge-shaped flutes, all of which have steep surfaces and vortex pits at their heads. Also, this specimen has a striking undercut facet beneath the high- velocity impact zone (0.75 x). J. Embossed vortex pit and helicoid scores, basal surface of sandstone ventifact, Big Sable Point, Michigan (2.5 x). K. Com- plexly eroded embossed pit on the top surface of the specimen in Figure J. This feature consists of an outer ring pit and a central boss which has undergone such profound erosion that a second ring pit has been eroded within the original boss, leaving only a very small ball-shaped boss within the inner ring (6x). L. Embossed vortex pit developed on top surface of wind-blasted halite block (about 50 x). M. Crescentic pit developed on left side of the wind-blasted margin of the same halite block as in "L" (about 30x). ventifacts, it was also possible to identify characteristics associated the flute pit, though it may become somewhat misshapen in wind with impact. Actually, identification of probable impact areas on shifts where the dominant air motion through it is negative flow. nearly all ventifacts is now possible. As a consequence, concen- However, the overall dominance of air motion in such pits is cyclic. trated study could be made of the areas of ventifacts which, in na- Many vortex pits have radial or helical or bench-type wall sculptur- ture, were under strong negative and secondary flow, the areas ing. The flute pit may became modified to a vortex pit as it deepens; most likely to become lineated. Studies of vorticity within pits a vortex pit, subject to shifting wind directions, may become mod- yielded extensive new data on the high degree of complexity of the ified to a flute pit. Flute pits commonly have burnished floors. vortex configuration and the intimate association of different kinds Where vorticity dominates, floors may lack burnish and mineral of flow and vorticity. fragments may remain sharp. A pit chain, a series of adjacent pits, can consist of vortex pits or flute pits or both. Such chains coalesce DEFINITIONS into lineations. Embossed pits are pits with elevated centers (Figs.
Details
-
File Typepdf
-
Upload Time-
-
Content LanguagesEnglish
-
Upload UserAnonymous/Not logged-in
-
File Pages18 Page
-
File Size-