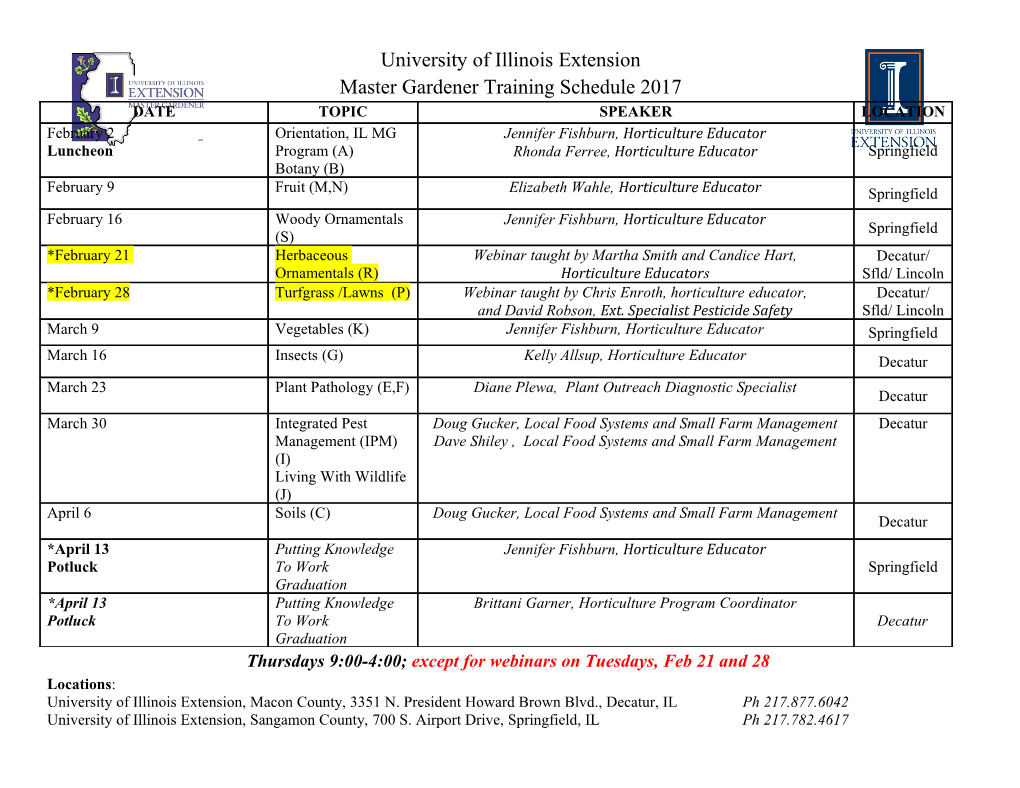
Stress modulation on the San Andreas fault by interseismic fault system interactions John P. Loveless* and Brendan J. Meade Department of Earth and Planetary Sciences, Harvard University, Cambridge, Massachusetts 02138, USA ABSTRACT of the SAF and a decrease on the MJ of 100 kPa, reducing the likelihood During the interseismic phase of the earthquake cycle, between of failure (Freed and Lin, 2002). Except following the largest earthquakes, large earthquakes, stress on faults evolves in response to elastic strain such as the Fort Tejon event, the contribution of postseismic deformation accumulation driven by tectonic plate motions. Because earthquake to the state of stress decays to apparently negligible levels within about a cycle processes induce non-local stress changes, the interseismic stress decade (Freed et al., 2007). accumulation rate on one fault is infl uenced by the behavior of all In contrast to the short time scales associated with coseismic and nearby faults. Using a geodetically constrained block model, we show postseismic stress changes, interseismic stress accumulation is gradual, that the total interseismic elastic strain fi eld generated by fault inter- yet characterizes the majority of each seismic cycle, building cumulatively actions within Southern California may increase stressing rates on the through the tens to hundreds of years between earthquakes and serving as Mojave and San Bernardino sections of the San Andreas fault within the primary mechanism driving future seismicity. On-fault stress accumu- the Big Bend region by as much as 38% relative to estimates from lates through the interseismic phase of the earthquake cycle when tem- isolated San Andreas models. Assuming steady fault system behav- porary frictional stability inhibits slip on the seismogenic fault interface, ior since the C.E. 1857 Fort Tejon earthquake, shear stress accumu- above a locking depth defi ned by the brittle-ductile transition zone at lated on these sections due only to interaction with faults other than 15–25 km depth, causing a buildup of elastic strain within the upper crust the San Andreas reaches 1 MPa, ~3 times larger than the coseismic (Fig. 1A; Savage and Burford, 1973). In the seismogenic layer this effect and postseismic stress changes induced by recent Southern Califor- is modeled using the slip defi cit (backslip) method (Savage, 1983), where nia earthquakes. Stress increases along Big Bend sections coincide the slip defi cit rate ranges from zero for a creeping fault to the long-term with the greatest earthquake frequency inferred from a 1500-yr-long paleoseismic record and may affect earthquake recurrence intervals within geometrically complex fault systems, including the sections of 0.6 the San Andreas fault closest to metropolitan Los Angeles. A ) 0 v / 0.4 INTRODUCTION v The San Andreas fault (SAF) in central-southern California accom- 0.2 modates as much as 35 mm/yr (Sieh and Jahns, 1984) of the 53 mm/yr of local relative motion between the Pacifi c and North America plates 0.0 (DeMets and Dixon, 1999). Historically, this motion has been expressed v /v seismically by the large C.E. 1812 M = 7.5 Wrightwood and 1857 M = −0.2 1 0 W W v /v 7.9 Fort Tejon earthquakes (Sieh et al., 1989). Further evidence for large- 2 0 −0.4 v /v magnitude earthquakes has been documented through paleoseismic stud- Strike−parallel velocity ( TOT 0 X /d ies revealing as many as 14 earthquakes averaging 3.2 m of slip per event 1 −0.6 X /d along the central-southern SAF since C.E. 550 (Weldon et al., 2004). 2 The spatial distribution of earthquake occurrence is not homogeneous τ /τ 1 max. 0.75 over this time interval: seismic events have more frequently ruptured the 1.0 τ /τ B 2 max. Δτ 0.50 Δτ Big Bend of the SAF, comprising the Mojave (MJ) and San Bernardino ) τ /τ TOT max. 0.25 (SB) sections within 50 km of metropolitan Los Angeles (Weldon et al., max. τ 0.8 X /d / 1 2004; Biasi and Weldon, 2009). During the past ~150 yr of relative seis- τ X /d 0 1 2 3 mic quiescence, interseismic earthquake cycle processes have continually 2 Fault separation (W/d ) 0.6 modulated shear and Coulomb stresses on the SAF while the seismically Δτ exposed population of greater Los Angeles has grown from fewer than 10,000 to more than 10 million (Stein and Hanks, 1998). 0.4 The total stress on a seismogenic fault surface results from the cumu- lative effects of coseismic, postseismic, and interseismic earthquake cycle Shear stress rate ( 0.2 processes. Elastic models of a 200-yr-long historical earthquake catalog W/d suggest abrupt coseismic Coulomb failure stress perturbations to 700 kPa 0.0 along the SAF (King et al., 1994; Freed et al., 2007). In the decade follow- −6 −4 −2 0 2 4 6 ing the 1992 MW = 7.3 Landers and 1999 MW = 7.1 Hector Mine earth- Strike−normal position (X/d ) quakes in the eastern California shear zone, postseismic deformation con- Figure 1. A: Interseismic fault-parallel surface velocities, v, due to slip trolled by viscoelastic relaxation processes in the lower crust and upper defi cit on two faults locked to depth d and spaced W = 2d. Slip defi cit mantle (Smith and Sandwell, 2006) resulted in a net 230–350 kPa increase rate is v0 on fault 1 (blue) and v0/2 on fault 2 (red). Total velocity due in Coulomb failure stress (CFS) on the SB and northern Indio (IN) section to slip defi cit on both faults is shown in black. B: Interseismic surface shear stress accumulation rates, τ. Difference between total stress ac- cumulation rate (black line) and self-stressing rate on fault 2 (red line), *Current address: Department of Geosciences, Smith College, Northamp- ΔΔττ, represents modulation of stress on one fault due to slip defi cit on ton, Massachusetts 01063, USA; E-mail: [email protected]. other. Inset shows decay of ΔΔττ with fault separation, W/d. © 2011 Geological Society of America. For permission to copy, contact Copyright Permissions, GSA, or [email protected]. GEOLOGY,Geology, November November 2011; 2011 v. 39; no. 11; p. 1035–1038; doi:10.1130/G32215.1; 3 fi gures; Data Repository item 2011305. 1035 A. GPS velocities B. Strike-slip rates m. 3 n. 3 j. -2 l. -4 a. 31 p. 1 q. 7 35˚N a. SAF Carrizo k. -2 o. 6 35˚N b. SAF WW-G b. 26 c. 16 r. 3 c. SAF Mojave d. SAF San Bernardino e. SAF Indio d. 10 f. SAF Imperial g. Imperial e. 24 N h. San Jacinto N h. 14 i. Elsinore j. White Wolf (WW) i. 4 k. Garlock (G) West 32.5˚N l. G Central f. 33 32.5˚N m. Panamint Valley n. Death Valley 100 km o. Lockhart 100 km g. 38 mm/yr p. Calico-Blackwater mm/yr q. Goldstone-Bullion 10 20 30 40 r. Ludlow −30 −15 0 15 30 122.5˚W 120˚W 117.5˚W 115˚W 122.5˚W 120˚W 117.5˚W 115˚W Figure 2. Block model constraints and results. A: Interseismic velocity fi eld (McClusky et al., 2001; Shen et al., 2003; Hammond and Thatcher, 2005; Williams et al., 2006; McCaffrey et al., 2007; Plate Boundary Observatory network velocity fi eld, http://pboweb.unavco.org) relative to stable North America. Arrow length is uniform; speed is denoted by color. GPS—global positioning system. B: Estimated strike-slip rates on block-bounding faults from our reference block model given by colored lines (right lateral is positive). Gray lines show block geometry, which is constructed by connecting faults with parameters specifi ed by the Southern California Earthquake Center Rectilinear Community Fault Model (Plesch et al., 2007) (black lines). SAF—San Andreas fault. Selected faults are labeled with average slip rate (rounded to near- est mm/yr). Full list of slip rates is in Table DR1 (see footnote 1). Figure constructed using Generic Mapping Tools (Wessel and Smith, 1998). fault slip rate for a locked fault. The interseismic stress accumulation rate strain accumulation due to interseismic locking of faults, and homoge- on an isolated fault is linearly proportional to its slip defi cit rate (Fig. 1B; neous strain rates within crustal microplates (McCaffrey, 2005). The block Okada, 1992). However, stresses generated by slip defi cit on one fault model fi ts the data with a mean residual velocity magnitude of 1.67 mm/yr extend throughout the upper crust (e.g., Hetland and Hager, 2006), decay- and simultaneously satisfi es far-fi eld Pacifi c−North America plate motion ing with distance as ~1/r for the near-fi eld two-dimensional case. Because constraints (DeMets and Dixon, 1999). of this non-local effect, the total stress accumulation on any fault segment Model results show that slip on the Carrizo (CZ) section of the SAF is the sum of the contributions from all active structures within the inter- currently accounts for as much as 60% of the 53 mm/yr of relative plate acting fault network (Fig. 1B). motion (DeMets and Dixon, 1999). However, the anastomosing geom- etry of the Southern California fault system partitions slip across mul- SAN ANDREAS FAULT STRESSING RATES tiple faults through the Big Bend region (Fig. 2B) and, as a consequence, To calculate current stressing rates on the southern-central SAF, we SAF strike-slip rates vary signifi cantly along strike: 31.2 ± 0.2 mm/yr use global positioning system (GPS) measurements of interseismic defor- on the CZ, 16.3 ± 0.8 mm/yr on the MJ, 10.2 ± 0.3 mm/yr on the SB, mation (Fig.
Details
-
File Typepdf
-
Upload Time-
-
Content LanguagesEnglish
-
Upload UserAnonymous/Not logged-in
-
File Pages4 Page
-
File Size-