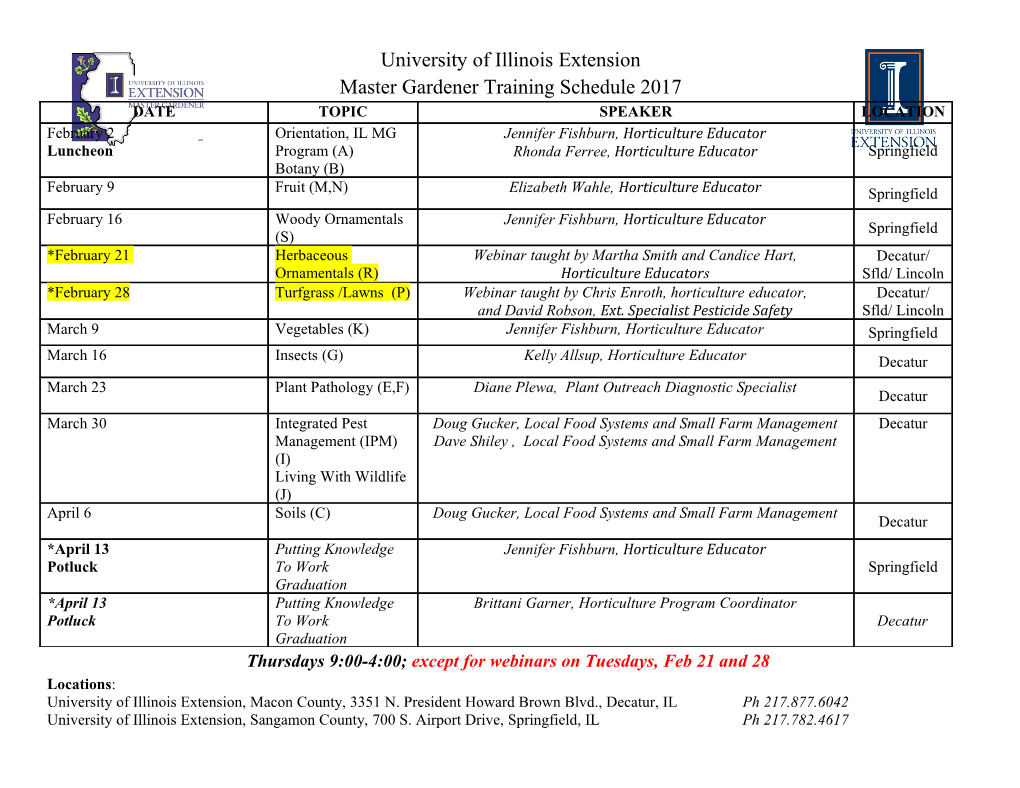
Report A Massive Expansion of Effector Genes Underlies Gall-Formation in the Wheat Pest Mayetiola destructor Highlights Authors d The plant-galling Mayetiola destructor genome is replete Chaoyang Zhao, with putative effector genes Lucio Navarro Escalante, ..., Jeffery J. Stuart, Stephen Richards d The SSGP-71 effector gene family is the largest known arthropod gene family Correspondence [email protected] d SSGP-71 genes encode E3-ubiquitin-ligase mimics d Two SSGP-71 genes elicit effector-triggered immunity in In Brief resistant wheat Zhao et al. reveal the effects of a genetic arms race on a plant-galling insect genome. One-eighth of the genes encode putative gall effectors, and the largest family encodes 426 secreted E3- ubiquitin-ligase-mimicking proteins necessary for galling and is similar to bacterial plant pathogen effectors, a remarkable example of molecular convergence. Zhao et al., 2015, Current Biology 25, 613–620 March 2, 2015 ª2015 Elsevier Ltd All rights reserved http://dx.doi.org/10.1016/j.cub.2014.12.057 Current Biology 25, 613–620, March 2, 2015 ª2015 Elsevier Ltd All rights reserved http://dx.doi.org/10.1016/j.cub.2014.12.057 Report A Massive Expansion of Effector Genes Underlies Gall-Formation in the Wheat Pest Mayetiola destructor Chaoyang Zhao,1,27 Lucio Navarro Escalante,1 Hang Chen,2,3 11Department of Biology, Lund University, 223 62 Lund, Thiago R. Benatti,1,28 Jiaxin Qu,4 Sanjay Chellapilla,5 Sweden Robert M. Waterhouse,6,7,8,9 David Wheeler,10 12The University of Chicago Bioinformatics Core, Biological Martin N. Andersson,11 Riyue Bao,12,13 Matthew Batterton,4 Sciences Division, Center for Research Informatics, The Susanta K. Behura,14 Kerstin P. Blankenburg,4 University of Chicago, Chicago, IL 60637, USA Doina Caragea,5,15 James C. Carolan,16 Marcus Coyle,4 13Department of Biological Sciences, Wayne State University, Mustapha El-Bouhssini,17 Liezl Francisco,4 Detroit, MI 48202, USA Markus Friedrich,13 Navdeep Gill,18 Tony Grace,5 14Department of Biological Sciences, University of Notre Cornelis J.P. Grimmelikhuijzen,19 Yi Han,4 Frank Hauser,19 Dame, IN 46556, USA Nicolae Herndon,5 Michael Holder,4 Panagiotis Ioannidis,6,7 15Department of Computer and Information Science, Kansas LaRonda Jackson,4 Mehwish Javaid,4 Shalini N. Jhangiani,4 State University, Manhattan, KS 66506, USA Alisha J. Johnson,20 Divya Kalra,5 Viktoriya Korchina,4 16Department of Biology, National University of Ireland Christie L. Kovar,4 Fremiet Lara,4 Sandra L. Lee,4 Maynooth, Maynooth, Ireland Xuming Liu,21 Christer Lo¨ fstedt,11 Robert Mata,4 17International Center for Agricultural Research in the Dry Tittu Mathew,4 Donna M. Muzny,4 Swapnil Nagar,5 Areas (ICARDA), Rabat BP 6299, Morocco Lynne V. Nazareth,4 Geoffrey Okwuonu,4 Fiona Ongeri,4 18Department of Botany, University of British Columbia, Lora Perales,4 Brittany F. Peterson,1 Ling-Ling Pu,4 Vancouver, BC V6T 1Z4, Canada Hugh M. Robertson,22 Brandon J. Schemerhorn,20 19Center for Functional and Comparative Insect Genomics, Steven E. Scherer,4 Jacob T. Shreve,1,29 DeNard Simmons,4 University of Copenhagen, Universitetsparken 15, 2100 Subhashree Subramanyam,23 Rebecca L. Thornton,4 Copenhagen, Denmark Kun Xue,24 George M. Weissenberger,4 20USDA-ARS, Department of Entomology, Purdue University, Christie E. Williams,25 Kim C. Worley,4 Dianhui Zhu,4 West Lafayette, IN 47907, USA Yiming Zhu,4 Marion O. Harris,26 Richard H. Shukle,20 21USDA-ARS, Department of Entomology, Kansas State John H. Werren,10 Evgeny M. Zdobnov,6,7 Ming-Shun Chen,21 University, Manhattan, KS 66056, USA Susan J. Brown,5 Jeffery J. Stuart,1 and Stephen Richards4,* 22Department of Entomology, University of Illinois at 1Department of Entomology, Purdue University, Urbana-Champaign, 320 Morrill Hall, 505 South Goodwin West Lafayette, IN 47097, USA Avenue, Urbana, IL 61801, USA 2Department of Entomology, Kansas State University, 23Department of Agronomy, Purdue University, West Manhattan, KS 66056, USA Lafayette, IN 47907, USA 3The Research Institute of Resource Insects, Chinese 24College of Life and Environment Sciences, Minzu University, Academy of Forestry, Bailongsi, Kunming 650224, People’s Beijing 100081, China Republic of China 25USDA-ARS, Department of Agronomy, Purdue University, 4Human Genome Sequencing Center, Department of West Lafayette, IN 47907 USA Molecular and Human Genetics, Baylor College of Medicine, 26Department of Entomology, North Dakota State University, One Baylor Plaza, Houston, TX 77030, USA Fargo, ND 58108, USA 5KSU Bioinformatics Center, Division of Biology, Kansas State University, Manhattan, KS 66506, USA 6Department of Genetic Medicine and Development, Summary University of Geneva Medical School, Rue Michel-Servet 1, 1211 Geneva, Switzerland Gall-forming arthropods are highly specialized herbivores 7Swiss Institute of Bioinformatics, Rue Michel-Servet 1, that, in combination with their hosts, produce extended phe- 1211 Geneva, Switzerland notypes with unique morphologies [1]. Many are economi- 8Computer Science and Artificial Intelligence Laboratory, cally important, and others have improved our understand- Massachusetts Institute of Technology, 32 Vassar Street, ing of ecology and adaptive radiation [2]. However, the Cambridge, MA 02139, USA mechanisms that these arthropods use to induce plant galls 9The Broad Institute of MIT and Harvard, 7 Cambridge Center, are poorly understood. We sequenced the genome of the Cambridge, MA 02142, USA Hessian fly (Mayetiola destructor; Diptera: Cecidomyiidae), 10Department of Biology, University of Rochester, Rochester, a plant parasitic gall midge and a pest of wheat (Triticum NY 14627, USA spp.), with the aim of identifying genic modifications that contribute to its plant-parasitic lifestyle. Among several adaptive modifications, we discovered an expansive reser- 27Present address: Department of Entomology, The Ohio State University, voir of potential effector proteins. Nearly 5% of the 20,163 Wooster, OH 44691, USA predicted gene models matched putative effector gene 28Present address: Centro de Tecnologia Canavieira, Sa˜ o Paulo 13400-970, transcripts present in the M. destructor larval salivary gland. Brazil Another 466 putative effectors were discovered among the 29 Present address: Department of Biochemistry and Molecular Biology and genes that have no sequence similarities in other organisms. the Huck Institutes of the Life Sciences, Pennsylvania State University, Uni- versity Park, PA 16802, USA The largest known arthropod gene family (family SSGP-71) *Correspondence: [email protected] was also discovered within the effector reservoir. SSGP-71 614 Figure 1. M. destructor and Its Damage to Wheat Seedlings (A) Adult female depositing eggs on a wheat leaf. Females use chemo- and mechanoreceptors to identify and orient themselves on host plants for oviposition. (B–F) Light-microscopic images of living 2-day-old first-instar larvae attacking individual epidermal cells using syringe-like mandibles and salivary secretions [14, 15]. a, antenna; b, supraesophageal ganglia; c, suboesophageal ganglia; fb, fat bodies; lm, labrum; m, mandible; mg, midgut; s, salivary gland. (B) After hatching, first-instar larvae move down the leaf and under the older leaves to attack the base of the seedling (bar, 0.5 mm). (C) View through the transparent dorsal cuticle of the larval thorax (bar, 0.1 mm). (D) A larva with its mouthparts attached to the leaf surface (bar, 10 mm). (E) Larva with its mandibles extended (bar, 5 mm). (F) The head of a larva (bar, 5 mm). (G) Near-isogenic wheat seedlings 10 days after M. destructor infestation. The resistant seedling (R) has three leaves (1–3) and shows no visible symptoms of M. destructor feeding. The susceptible seedling (S) has only two leaves and will grow no further. Note the position of the ligule of the first leaf on both plants (arrows) and how the second leaf blade of the S plant fails to grow past this point. proteins lack sequence homologies to other proteins, but assembled with an existing fingerprinted contig (FPC)-based their structures resemble both ubiquitin E3 ligases in plants contig map, consisting of end-sequenced M. destructor and E3-ligase-mimicking effectors in plant pathogenic bac- bacterial artificial chromosomes (BACs) [16]. This allowed us teria. SSGP-71 proteins and wheat Skp proteins interact to position 59% of the genome sequence on the four in vivo. Mutations in different SSGP-71 genes avoid the M. destructor polytene chromosomes. The final genome as- effector-triggered immunity that is directed by the wheat sembly (NCBI BioProject PRJNA45867) contains 153 Mb of resistance genes H6 and H9. Results point to effectors as assembled contig sequence with a 14 kb contig N50 length, the agents responsible for arthropod-induced plant gall across an extent of 186 Mb (including gaps between contigs) formation. and a 756 kb scaffold N50 length. To assess the completeness of the assembly, we examined Results and Discussion the M. destructor genome using benchmarking sets of univer- sal single-copy orthologs (BUSCOs). When genome assembly Recent investigations suggest that ‘‘effector’’ proteins, struc- is complete, BUSCOs from the appropriate phylogenetic clade turally undefined proteins that suppress host defense and are found as single copies in the newly sequenced genome modulate host cell activities [3–6], are involved in the formation [17]. Using the monarch butterfly, Danaus plexippus, as the of insect-induced plant galls [7–10]. Other investigations outgroup reference species, we compared the M. destructor have demonstrated that genomic sequencing can reveal the genome assembly to two species of Drosophila and two effector repertoires of important plant parasites [11–13]. We mosquitoes. M. destructor has slightly more missing or dupli- therefore sequenced the genome of the wheat-galling midge cated BUSCOs than Drosophila melanogaster and Anopheles Mayetiola destructor (Figure 1), to search for effectors and gambiae, but fewer than Culex quinquefasciatus. In addition, other modifications that permit the insect to live as a gall-form- M. destructor BUSCO length recovery was similar to that of ing plant parasite. An inbred strain selected from the avirulent the other dipteran genomes.
Details
-
File Typepdf
-
Upload Time-
-
Content LanguagesEnglish
-
Upload UserAnonymous/Not logged-in
-
File Pages9 Page
-
File Size-