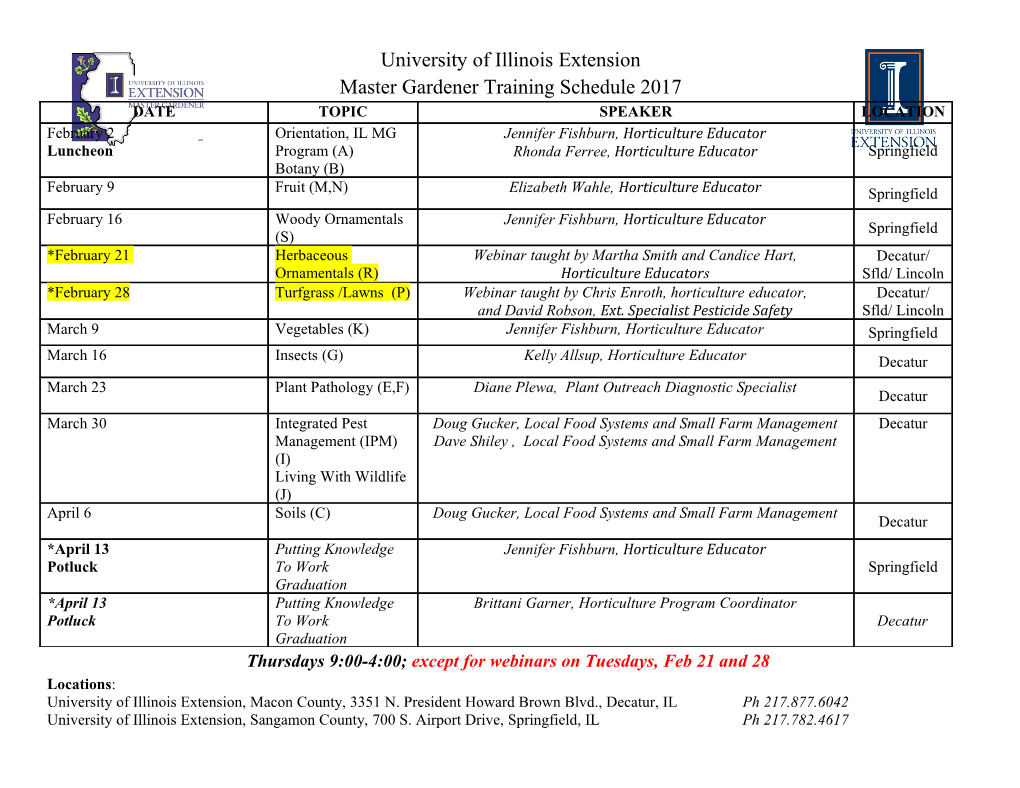
Can xenon in water inhibit ice growth? Molecular dynamics of phase transitions in water–Xe system Vasilii I. Artyukhov,1, a) Alexander Yu. Pulver,2 Alex Peregudov,2 and Igor Artyuhov2, b) 1)Department of Materials Science and NanoEngineering, Rice University, Houston, Texas 77005 2)Institute of Biology of Aging, Moscow, 125284 Russia Motivated by recent experiments showing the promise of noble gases as cryoprotectants, we perform molecular dy- namics modeling of phase transitions in water with xenon under cooling. We follow the structure and dynamics of xenon water solution as a function of temperature. Homogeneous nucleation of clathrate hydrate phase is observed and characterized. As the temperature is further reduced we observe hints of dissociation of clathrate due to stronger hydrophobic hydration, pointing towards a possible instability of clathrate at cryogenic temperatures and conversion to an amorphous phase comprised of “xenon + hydration shell” Xe·(H2O)21.5 clusters. Simulations of ice–xenon solution interface in equilibrium and during ice growth reveal the effects of xenon on the ice–liquid interface, where adsorbed xenon causes roughening of ice surface but does not preferentially form clathrate. These results provide evidence against the ice-blocker mechanism of xenon cryoprotection. I. INTRODUCTION (iv) Accumulation of xenon in lipid membranes.24,25 (v) Protection against protein thermal denaturation.17 Ever since the original deciphering of clathrate hydrate structures by Claussen, Pauling, and others,1–4 researchers In the present molecular dynamics study we focus on the have been fascinated by the interactions of hydrophobic gases “nonbiochemical” mechanisms (i–iii) while the interaction with water producing these beautiful crystal structures. Natu- of xenon with biological membranes and proteins is left for ral gas hydrates are a potential source of energy5 and problem follow-up studies. First we investigate the structure and prop- in fossil fuel transport;6 clathrates could play a role in global erties of homogeneous xenon solution and its behavior under 7 8 climate change and be used in CO2 sequestration. Clathrate cooling below the freezing temperature of water. We observe hydrates were famously proposed as a molecular explanation the formation of clathrate phase, with a complex dependence of general anaesthesia.9 on the concentration and temperature of solution, and a sig- Xenon, a noble gas that readily forms a clathrate hydrate, nature of another metastable amorphous phase upon deep su- manifests distinct physiological actions despite its chemical percooling. Then we study the process of ice growth in the inertness. Owing to its strong anesthetic effect,10 xenon is presence of dissolved xenon, observing preferential adsorp- considered to be the best (though expensive) inhalation anes- tion of xenon on the ice surface but no preferential nucleation thetic to date and is officially approved for application as that could have caused an ice-blocking effect. such in Germany and Russia. Xenon has an antidepressant effect (presumably due to its anti-NMDA11 action) and is used to treat alcoholism and drug addiction.12 Xenon shows II. COMPUTATIONAL DETAILS a protective effect on various organs subject to ischaemia– 11,13,14 reperfusion injury (presumably due to its anti-NMDA A. Simulation method and models effect and interference with many aspects of intracellular Ca2+ 15 homeostasis ). For xenon, we used Lennard–Jones interaction An important potential application of xenon is its cryopro- 26 16–19 parameters that were previously successfully used to tective action. In order to understand this phenomenon, study hydrophobic hydration of xenon by Paschek:27 the physicochemical basis of interaction of xenon with liquid s = 3:975, e=kB = 214:7K. To simulate water, we used water, ice and biological molecules must be understood at the the TIP5P five-site rigid model.28 Cross-species interaction microscopic level. The mechanisms that xenon’s antifreeze parameters were derived using the geometrical mean com- effect could be ascribed to include: bination rule. TIP5P was chosen to represent water because arXiv:1407.3226v1 [cond-mat.soft] 11 Jul 2014 (i) Trapping of water in clathrate hydrate in competition it has pronounced directional hydrogen bonding thanks to with ice crystals formation (akin to traditional cryopro- its lone-pair virtual sites, leading to stronger tetrahedral tectants such as glycerol and DMSO). structuring of water, which is important here because of our (ii) Vitrification20 of xenon solution. interest in the processes of hydrophobic hydration that is cru- 27 (iii) Ice-blocker action via kinetic suppression of ice cially dependent on the local ordering of water molecules 29 growth (similar to biological antifreeze proteins21,22) by (especially at low temperatures ), and crystal formation. clathrate hydrate forming on ice crystal surface.23 The parametrization of the TIP5P model was done over a wide range of temperatures and pressures,28 and it correctly reproduces both the anomalous density maximum of water at 4 °C as well as the melting temperature of ice Ih (TM = 275 K a)Electronic mail: [email protected] as observed in the present work and in earlier studies30–33). b)Electronic mail: [email protected] Although the thermodynamically most stable ice polymorph 2 of radial distribution function (RDF) and mean-square dis- TABLE I. Duration of simulation runs for homogeneous systems (ns) placement (MSD) plots; at the lowest concentration of 1%, T (K) 280 270 265 260 255 250 though the spatial configurations of xenon atoms were written 1% 80 80 50 100 100 >100 out as frequently as once every 100 fs (more than sufficient, given the typical timescale of xenon motion of ∼1 ps, as ob- 2% 50 50 50 100 100 >100 served below), RDF plots still contain noticeable statistical 3% 30 30 50 100 100 >100 noise. 4% 30 30 50 100 100 >100 Two-phase solid-liquid equilibrium simulations were per- 5% 30 50 100 100 100 >100 formed with a 1010 ice Ih slab, since the prism plane of ice grows faster than basal and hence is the most relevant from the viewpoint of both pure physics44 and biological is erroneously predicted by TIP5P to be ice II,30 and the applications.21 The size of the slab was 5:3 × 5:8 nm, and proton-ordered ice Ih structure has lower predicted free the thickness was 3.07 nm, corresponding to four nonprimi- energy than the (correct) disordered ice I,33 we still believe tive crystal cells, or eight water molecule bilayers (see figures TIP5P to be the most reliable choice of water model for our below); the total number of water molecules in the slab was purposes. 3072. Initial coordinates for ice molecules were taken from Molecular dynamics simulations were carried out using the work of Buch et al.45 The liquid layers were taken ap- the GROMACS package.34 The simulation time step was 2 fs. proximately twice as thick as the slab (6.1 nm) to accommo- Coulomb and Lennard-Jones interactions were truncated at a date enough xenon molecules and to allow us to study longer- distance of 0.9 nm (as in the original TIP5P parametrization). range effects of ice on the structure of the solution. Liquid and Long-range Coulomb interactions were calculated using the solid phases preequilibrated at 275 K were brought in contact Particle-Mesh Ewald method,35,36 and a standard long-range and relaxed using steepest descent energy minimization with dispersion correction term was applied.37 For liquid systems, a maximum force criterion of 100 (kJ/mol)/nm, which was preequilibration was done using the Berendsen thermostat,38 found to be sufficient to remove any bad molecular contacts and production runs were performed using the Nosé–Hoover at the interface while still ensuring that atomic coordinates thermostat39,40 with a 0.5 ps time constant and the Nosé– still correspond well to the 270–275 K temperature of subse- Parrinello–Rahman barostat41,42 with a 2.0 ps time constant. quent production runs, thus providing smooth continuation of For two-phase systems, production runs used the velocity molecular dynamics. 43 38 rescaling thermostat and the Berendsen barostat with a 0.5 The results reported herein were mostly produced at a sim- ps time constant for both, to accommodate the total energy and ulated pressure of 1 atm irrespective of xenon concentration volume change during phase transition. in the systems. While xenon clathrate hydrate is stable under these conditions or close (its experimental dissociation pres- sure value is 2.5 bar at 5 °C, 1.5 bar at 0 °C, and 1 bar at B. System preparation and simulation protocols -3.6 °C46), these concentrations are considerably above equi- librium at a pressure of 1 atm, which may create an excessive Liquid systems were prepared by randomly inserting xenon driving force for clathrate formation. Extrapolation of em- molecules into a preequilibrated 500-molecule water box to pirical xenon solubility data47 to our temperature range sug- produce a desired xenon concentration of 1, 2, 3, 4, or 5% gests that our concentrations should correspond to “equilib- mol.Xe/mol.H2O (meaning 100, 80, 60, 40, and 20 water rium” (barring formation of solid phases) pressures on the or- molecules per xenon, respectively). The 5% concentration der of tens to few hundred atmospheres, which is, in fact, a was chosen as the upper boundary since it still, in princi- much milder driving force than used in most if not all recent ple, allows each xenon molecule to have its separate hydration MD studies on clathrate formation. We never observed sepa- shell (which contains about 20 molecules); at higher concen- ration of xenon gas phase in our simulations; in contrast, such trations, there would necessarily be insufficient water to sol- separation was observed to occur spontaneously in molecular vate each xenon molecule, casting doubt on our treatment of dynamics simulations of methane hydrate at 100 MPa;48 note the system as a liquid solution.
Details
-
File Typepdf
-
Upload Time-
-
Content LanguagesEnglish
-
Upload UserAnonymous/Not logged-in
-
File Pages11 Page
-
File Size-