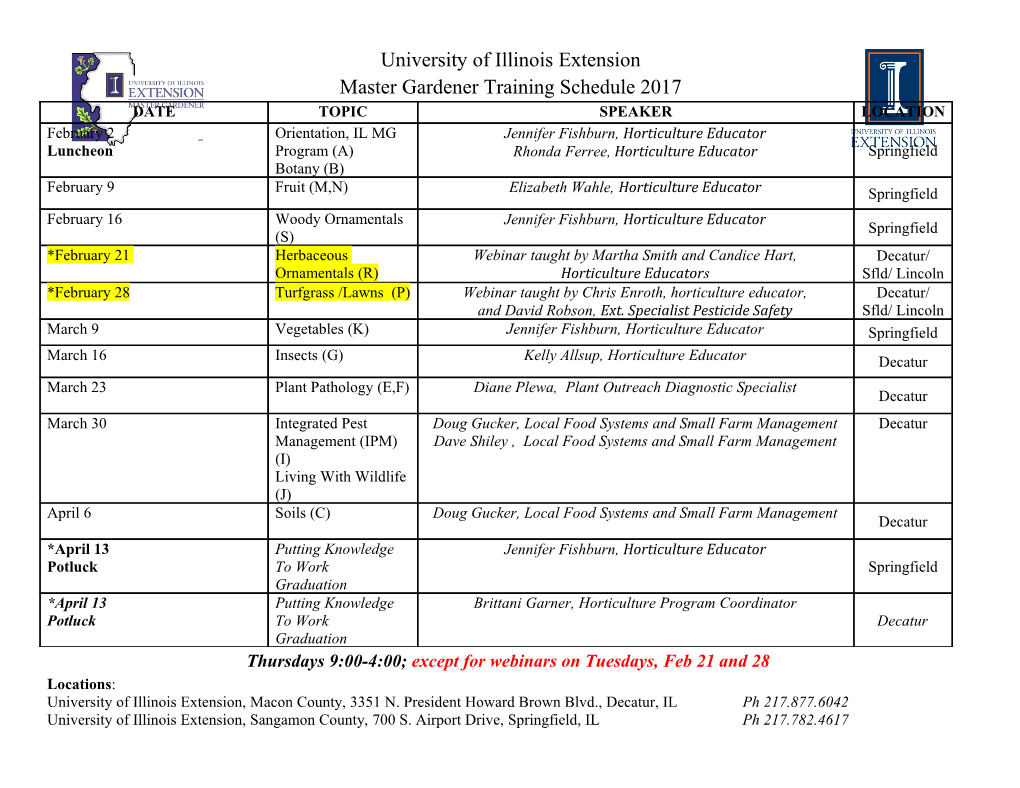
Journal of Experimental Botany, Vol. 56, No. 416, pp. 1449–1462, June 2005 doi:10.1093/jxb/eri161 Advance Access publication 29 April, 2005 FOCUS PAPER Chloroplasts as source and target of cellular redox regulation: a discussion on chloroplast redox signals in the context of plant physiology Margarete Baier* and Karl-Josef Dietz Biochemistry and Physiology of Plants, University of Bielefeld, D-33501 Bielefeld, Germany Received 28 January 2005; Accepted 15 March 2005 Abstract Key words: Abscisic acid, antioxidants, chloroplast, gene expression, oxolipin, peroxiredoxin, photosynthesis, redox During the evolution of plants, chloroplasts have lost regulation, signalling, stress. the exclusive genetic control over redox regulation and antioxidant gene expression. Together with many other genes, all genes encoding antioxidant enzymes and enzymes involved in the biosynthesis of low molecular Introduction weight antioxidants were transferred to the nucleus. On the other hand, photosynthesis bears a high risk for In plants, photosynthesis generates redox intermediates photo-oxidative damage. Concomitantly, an intricate with extraordinarily negative redox potentials. Light-driven network for mutual regulation by anthero- and retro- electron transport transfers electrons from the acceptor site grade signals has emerged to co-ordinate the activities of photosystem I (Em < ÿ900 mV) to various acceptors of the different genetic and metabolic compartments. A including oxygen (Em = 815 mV; Blankenship, 2002). The major focus of recent research in chloroplast regula- redox intermediates cover an exceptionally wide range of tion addressed the mechanisms of redox sensing and mid-point redox potentials (Dietz, 2003) with a significant signal transmission, the identification of regulatory risk for electron transfer to oxygen and other appropriate targets, and the understanding of adaptation mech- targets. Among the best known examples for chloroplast anisms. In addition to redox signals communicated redox chemistry is the direct electron transfer from reduced through signalling cascades also used in pathogen ferredoxin to O2 in the so-called Mehler reaction (Mehler, and wounding responses, specific chloroplast signals 1951). The superoxide radicals formed can be quickly control nuclear gene expression. Signalling pathways converted into H2O2 and highly reactive hydroxyl radicals c are triggered by the redox state of the plastoquinone (HO ) (Elstner, 1990). Together with reactive oxygen pool, the thioredoxin system, and the acceptor avail- species (ROS) generated by other sources, they are a con- ability at photosystem I, in addition to control by tinuous threat to cellular constituents for uncontrolled oxolipins, tetrapyrroles, carbohydrates, and abscisic oxidation. acid. The signalling function is discussed in the con- In the context of (i) the physiologically bivalent oxygen text of regulatory circuitries that control the expression chemistry, (ii) the demand for reductive power, and (iii) the of antioxidant enzymes and redox modulators, demon- peril of excess photosynthetic electron pressure, chloro- strating the principal role of chloroplasts as the source plasts are prone to oxidative damage like no other organelle and target of redox regulation. (Foyer et al., 1997). Consequently, photosynthetic organ- isms have evolved defence mechanisms to control the redox poise of the electron transport chain and the redox envir- onment of the stroma. They range from the suppression of * To whom correspondence should be addressed. Fax: +49 (0)521 106 6039. E-mail: [email protected] Abbreviations: 2CPA, 2-Cys peroxiredoxin A; ABA, abscisic acid; DBMIB, 3-methyl-6-isopropyl-p-benzoquinone; DCMU, 3-(39,49-dichlorophenyl-)1,1- dimethyl urea; DOX, fatty acid dioxygenase; Em, mid-point potential; LOX, lipoxygenase; MAPK(K)(K), mitogen activated protein kinase (kinase) (kinase); NDH, NAD(P)H dehydrogenase; PET, photosynthetic electron transport; PQ, plastoquinone; Prx, peroxiredoxin; ROS, reactive oxygen species; Rubisco, ribulose-1,5-bisphosphate carboxylase oxygenase. ª The Author [2005]. Published by Oxford University Press [on behalf of the Society for Experimental Biology]. All rights reserved. For Permissions, please e-mail: [email protected] 1450 Baier and Dietz ROS generation and avoidance of uncontrolled oxidation of essential biomolecules by accumulating high concentra- tions of low-molecular weight antioxidants to repair mech- anisms by reduction and de novo synthesis of damaged molecules. In the illuminated chloroplast, the reduction energy for the regeneration of oxidized metabolites is mainly taken from the photosynthetic electron transport (PET). Depending on the photo-oxidative strain, up to almost 100% of the photosynthetically transported elec- trons can be diverted into the antioxidant defence system (Asada, 2000). In the dark, when the strong reductive power of photosynthesis is missing, the redox environment of chloroplasts probably adjusts to redox potentials similar to those measured in the cytoplasm of heterotrophic cells (i.e. below ÿ300 mV to approximately ÿ240 mV; Dietz, 2003). Under these conditions, chloroplasts stabilize their redox poise by metabolization of starch and import of Fig. 1. The cytosolic antioxidant system shields the nucleus from chloro- plast ROS signals. Photosynthetic ROS signals and redox imbalances are reduction energy. buffered by cytosolic antioxidants. Whether they reach the nucleus Studies with various transgenic plant lines and mutants depends on the rates of ROS-formation and the strength of the cytosolic demonstrated the importance of a high antioxidant cap- antioxidant system. In contrast, a non-redox active second messenger can pass the cytosol without loss of function. acity as realized by low-molecular weight antioxidants (Pastori et al., 2003; Ball et al., 2004) and antioxidant enzymes (Willekens et al., 1997; Baier et al., 2000; may have additional functions, for example, as a metabolic Davletova et al., 2005). The antioxidant defence system re- intermediate. sponds to redox imbalances. The pool size of low molecu- lar weight antioxidants and the expression of chloroplast antioxidant enzymes increases if the photo-oxidative strain Redox coupling on the system is high (Foyer et al., 1997). Interestingly, oxidizing conditions correlate with a high reduction state, All redox active compounds together define the cellular since, as outlined above, excess electrons are transferred redox poise of a cell (Schafer and Buettner, 2001). In to O2 that, in turn, abstract electrons from organic targets. addition to the redox energetics defined by the mid-point This apparent contradiction (‘high reducing activity potentials and the concentrations of the various antioxi- results in oxidizing conditions’) will be important for dants, the redox state of a particular compound is under the understanding redox regulation, as for example in the control of kinetic constraints defining the reaction constants. peroxiredoxin function, in the context of photosynthesis Enzymes lower the kinetic barriers and couple redox pairs. (see below). However, all chloroplast antioxidant enzymes Recycling of oxidized intermediates, as well as regeneration and all enzymes involved in the biosynthesis of low- of the reductants, affect the redox state of specific redox molecular-weight antioxidants are nuclear encoded. The pairs (Dietz, 2003). Different drainage rates of electrons spatial separation of expression and function demands for from antioxidants and weak enzymatic linkages can un- signal transduction over the chloroplast envelope. Devia- couple the redox states of individual components of the tions from regular redox homeostasis can be sensed in the antioxidant network, as has been observed for ascorbate and chloroplast and transmitted to the nucleus by retrograde glutathione. While specific oxidation of the glutathione pool signalling cascades. Alternatively, redox imbalances in the took place in catalase-deficient barley (Willekens et al., chloroplast could be transmitted into the cytoplasm by 1997), reduced 2-Cys peroxiredoxin levels led preferen- metabolic coupling (Fig. 1). The sensor then resides in tially to the oxidation of the ascorbate pool in Arabidopsis the cytoplasm. Transmission of redox signals suffers thaliana (Baier et al., 2000). The favoured oxidation of from the drawback that cytoplasmic redox homeostasis glutathione in the catalase-lines is proposed to be caused by and antioxidant defence will strongly damp the spreading higher cytosolic dehydroascorbate reductase activity rather signal. than glutathione reductase activity, while the redox shift in Another terminology to be clarified is the distinction of the ascorbate pool in the peroxiredoxin antisense plants signalling and regulation. Both terms are used with a broad might be caused by insufficient dehydroascorbate reductase meaning here. Regulation describes any adjustment of activity in the chloroplasts (Noctor et al., 2000). For cellular activity, i.e. of enzymes or transcription factors, while redox homeostasis and redox metabolism, the nature of signalling refers to any transport of information from one a particular antioxidant employed in a specific detoxifica- site to another via a signalling molecule that, by itself, tion reaction and, within limits, the relative redox state of an Chloroplast redox regulation 1451 individual antioxidant may be of minor importance. How- Sensing the cellular redox poise ever, deviations from
Details
-
File Typepdf
-
Upload Time-
-
Content LanguagesEnglish
-
Upload UserAnonymous/Not logged-in
-
File Pages14 Page
-
File Size-