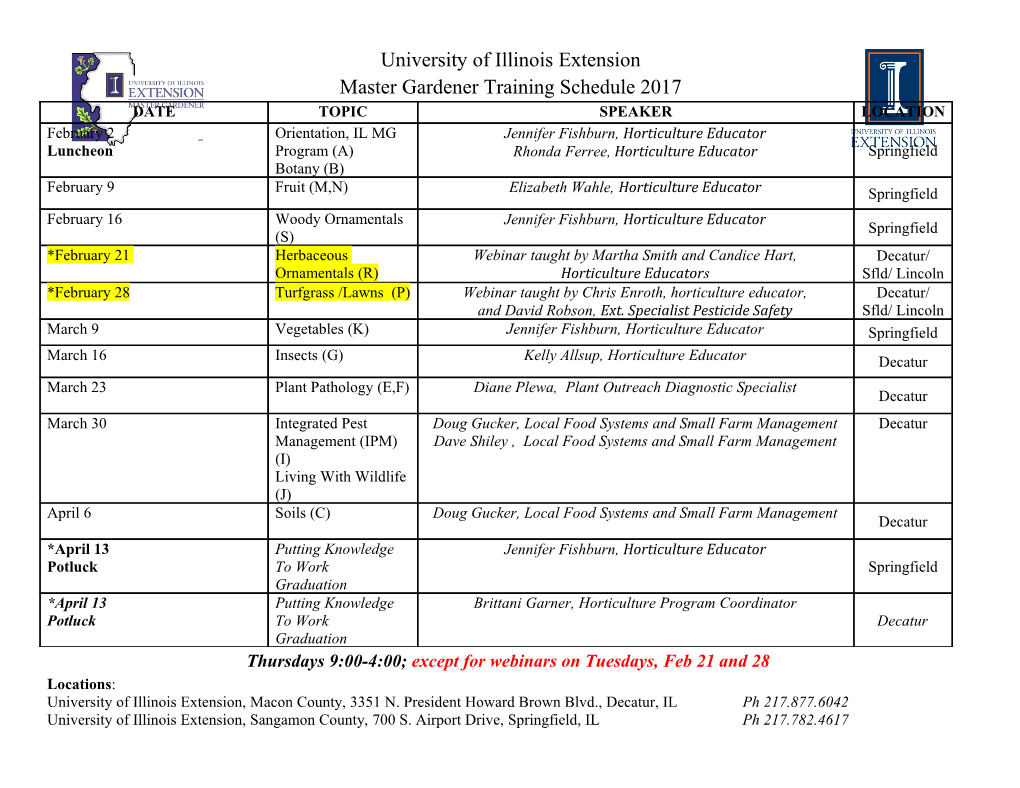
RESOURCE https://doi.org/10.1038/s41556-018-0093-4 Reprogramming of H3K9me3-dependent heterochromatin during mammalian embryo development Chenfei Wang 1,2, Xiaoyu Liu1,2, Yawei Gao 1,2*, Lei Yang1,2, Chong Li1, Wenqiang Liu1, Chuan Chen1, Xiaochen Kou1, Yanhong Zhao1, Jiayu Chen1, Yixuan Wang1, Rongrong Le1, Hong Wang1, Tao Duan1, Yong Zhang 1* and Shaorong Gao 1* H3K9me3-dependent heterochromatin is a major barrier of cell fate changes that must be reprogrammed after fertilization. However, the molecular details of these events are lacking in early embryos. Here, we map the genome-wide distribution of H3K9me3 modifications in mouse early embryos. We find that H3K9me3 exhibits distinct dynamic features in promoters and long terminal repeats (LTRs). Both parental genomes undergo large-scale H3K9me3 reestablishment after fertilization, and the imbalance in parental H3K9me3 signals lasts until blastocyst. The rebuilding of H3K9me3 on LTRs is involved in silencing their active transcription triggered by DNA demethylation. We identify that Chaf1a is essential for the establishment of H3K9me3 on LTRs and subsequent transcriptional repression. Finally, we find that lineage-specific H3K9me3 is established in post-implanta- tion embryos. In summary, our data demonstrate that H3K9me3-dependent heterochromatin undergoes dramatic reprogram- ming during early embryonic development and provide valuable resources for further exploration of the epigenetic mechanism in early embryos. ammals develop from fertilized eggs, which confer distinct To address the above questions, we performed comprehensive epigenetic modifications from both parental genomes. analyses of H3K9me3-dependent heterochromatin dynamics in MDuring fertilization, these epigenetic modifications pre-implantation mouse embryos as well as in differentiated embry- undergo extensive reprogramming for compatibility with embryonic onic tissues after implantation using a recently published ultra- totipotency1,2. Specifically, the constitutive heterochromatin marker low-input chromatin immunoprecipitation followed by sequencing histone 3 lysine 9 trimethylation (H3K9me3) is rapidly remodelled (ULI-NChIP–seq) method19,20. Based on this high-resolution map, to create a chromatin environment that is compatible with cellular we investigated the reprogramming of H3K9me3-dependent het- reprogramming and plasticity3. Meanwhile, H3K9me3-dependent erochromatin during mouse early embryonic development and also heterochromatin is also involved in embryonic gene regulation and explored the molecular mechanism of LTR silencing by H3K9me3- imprinted X inactivation during early embryonic development4–6. dependent heterochromatin in pre-implantation embryos. Depletion of the genes encoding H3K9me3-related chromatin fac- tors, such as Ehmt2 and Trim28, causes improper gene expression Results and embryonic lethality7,8. However, previous studies investigat- Genome-wide profiling of H3K9me3 in mouse gametes and ing the H3K9me3 dynamics in vivo based on immunofluorescence early embryos. We generated H3K9me3 profiles of mouse meta- staining have been limited by the imaging resolution and lack of an phase II (MII) oocytes, sperm and embryos from the zygote stage overarching regulatory framework9–11. Hence, genome-wide analy- (pronuclear stage 3 (PN3)) to embryonic day 8.5 (E8.5), mESCs ses of H3K9me3-dependent heterochromatin dynamics in pre- and mouse trophoblast stem cells (TSCs). Embryos at the blasto- implantation embryos are sorely needed. cyst stage were separated into the inner cell mass (ICM) and the The mouse genome is extensively demethylated during early trophectoderm, and post-implantation embryos were separated embryonic development12,13, and a large fraction of long termi- into epiblast and extraembryonic tissue (Fig. 1a,b). RNA sequenc- nal repeats (LTRs) become hypomethylated and are actively tran- ing (RNA-seq) and whole-genome bisulfite sequencing (WGBS), as scribed, which are crucial for normal development14,15. These LTRs well as ChIP–seq of the H3K4me3 and H3K27me3 levels (includ- must be properly regulated in later developmental stages to maintain ing some published data20) for each corresponding stage, were also genome stability16. Studies in mouse embryonic stem cells (mESCs) performed for comprehensive analysis (Supplementary Table 1). We have suggested that multiple H3K9me3 modifiers, such as Suv39h1/ found that the global H3K9me3 enrichment in ESCs generated from h2 and ERG-associated protein with SET domain (ESET) complexes, 500 cells was consistent with the ENCODE data from 10–20 mil- are responsible for retrovirus silencing by establishing H3K9me3 lion cells (Supplementary Fig. 1a). Moreover, the two replicates of modifications17,18. However, little is known about the involvement H3K9me3 for each embryonic stage had Pearson's correlation coef- of H3K9me3-dependent heterochromatin in LTR silencing during ficients > 0.9, indicating the high reproducibility of the H3K9me3 development in vivo. data (Supplementary Fig. 1b). 1Clinical and Translational Research Center of Shanghai First Maternity and Infant Hospital, Shanghai Key Laboratory of Signaling and Disease Research, School of Life Sciences and Technology, Tongji University, Shanghai, China. 2These authors contributed equally: Chenfei Wang, Xiaoyu Liu, Yawei Gao and Lei Yang. *e-mail: [email protected]; [email protected]; [email protected] NATURE CELL BIOLOgy | www.nature.com/naturecellbiology © 2018 Macmillan Publishers Limited, part of Springer Nature. All rights reserved. RESOURCE NATURE CELL BIOLOGY a MII E6.5 E7.5 Zygote2 cell 4 cell 8 cell Morula Blastocyst TE Exe Exe Sperm ICM Epi Epi b c Promoter region 100 kb mm9 Scale 10 kb mm9 Scale 3 H3K4me3 Chr7: Chr2: 174,130,000 11,700,000 11,800,000 2 H3K27me3 5 - MII 5 - 1 MII 0 _ H3K9me3 0 _ 5 - 0 5 - Sperm Sperm 0 _ −1 0 _ 5 - 5 - Zygote Zygote 0 _ 0 _ SINE region 5 - 5 - ) 2 cell 2 1.0 2 cell 0 _ 0 _ 5 - 5 - 4 cell 4 cell 0 _ 0.0 0 _ 5 - 5 - 8 cell 8 cell 0 _ 0 _ 5 - −1.0 5 - Morula Morula 0 _ 0 _ 5 - 5 - ICM ICM 0 _ 0 _ LINE region 5 - 5 - H3K9me3 TE TE H3K9me3 0 _ 0 _ 5 - 0.0 5 - E6.5 Epi E6.5 Epi 0 _ −1.0 0 _ 5 - 5 - E6.5 Exe E6.5 Exe 0 _ −2.0 0 _ 5 - 5 - E7.5 Epi Observed/expected ration (log E7.5 Epi 0 _ 0 _ 5 - 5 - E7.5 Exe LTR region E7.5 Exe 0 _ 0 _ 5 - 5 - E8.5 embryo 2.0 E8.5 embryo 0 _ 0 _ 5 - 1.0 5 - Encode.ESC Encode.ESC 0 _ 0 _ 5 - 0.0 5 - ESC ESC 0 _ −1.0 0 _ 5 - 5 - TSC TSC 0 _ 0 _ TE MII ICM Nespas Gnas Zscan4c Zscan4d 2 cell 4 cell 8 cell ESC TSC Zygote Morula LTR90B LTR E6.5 EpiE6.5 ExeE7.5 EpiE7.5 Exe E8.5 embryro de1.0 f 100 6 ) 5 80 0.5 ) 3 4 60 2 0.0 3 40 2 H3K9me3 domains (K) 1 −0.5 H3K9me3 domains (K) Number of LTRs marked by 20 Number of genes marked by 1 Fraction of total H3K9me3 regions (1 kb H3K9me3-marked regions compared to the genome (% 0 −1.0 0 0 TE TE TE TE MII ICM ICM MII ICM MII ICM 2 cel4l cel8l cell ESCTSC 2 cell 4 cell8 cell 2 cell4 cell8 cell ESC TSC 2 cell4 cell8 cell ESCTSC SpermZygote Morula Zygote Morula SperZygotm e Morula SperZygotm e Morula E6.5 E6.5ExeE7.5 EpiE7.5 Epi Exe E6.5E6.5 Epi ExeE7.5E7.5 Epi Exe E6.5E6.5 Epi ExeE7.5E7.5 Epi Exe E6.5E6.5 Epi E7.5ExeE7.5 Epi Exe E8.5 embryo E8.5 embryo E8.5 embryo E8.5 embryo Fig. 1 | Genome-wide profiling of H3K9me3 in mouse gametes and early embryos. a, Schematic showing the preparation of mouse embryos for genome- wide ChIP–seq analysis of H3K9me3. Samples of MII oocytes and sperm; zygotes, 2-cell-, 4-cell-, 8-cell- and morula-stage embryos; the ICM and the trophectoderm (TE) of E3.5 blastocysts; epiblast (Epi) and extraembryonic ectoderm (Exe) of E6.5 and E7.5 of post-implantation embryos are analysed. b, The UCSC genome browser view of H3K9me3 signals around Zscan4d (right panel) and Gnas (left panel) in mouse gametes, early embryos, mESCs (from either this study or from ENCODE) and mouse TSCs. Signals represent the log2-transformed H3K9me3/input ratio. Chr, chromosome. c, Bar plots show the enrichment of H3K9me3, H3K4me3 and H3K27me3 in promoter, SINE, LINE and LTR regions. d, Graph showing the percentage of genomic regions covered by H3K9me3 peaks. e, Graph showing the fraction of established and disappeared H3K9me3 domains during embryonic development. f, Graph showing the number of H3K9me3-marked LTR regions (left panel) and promoter regions (right panel) during embryonic development. In b–f, ChIP–seq for H3K9me3 was performed two times for each indicated stage, except for TSCs (three times) and E7.5 epiblast tissue (four times), and data shown represent the average values for each stage. In c, H3K4me3 and H3K27me3 ChIP–seq data for MII oocyte, sperm, 2-cell-, 4-cell-, 8-cell- and morula-stage embryos, E3.5 ICM, E3.5 trophectoderm and TSCs were from our previous publication (GSE73952)20 and others were derived from this study: H3K4me3 ChIP–seq was performed two times except for E7.5 epiblast and E7.5 extraembryonic tissues (three times), and H3K27me3 ChIP–seq was performed three times except for E7.5 epiblast and ESCs (two times). To identify H3K9me3-enriched domains during early embryonic chromatin using sequencing (ATAC-seq) signals at promoter regions development, we performed peak calling analysis using model-based and positively correlated with traditional repressive modifications analysis for ChIP-seq (MACS)21. We first examined the distribution (Supplementary Fig. 1d,e), reflecting the compatibility between of the H3K9me3 domains in early embryos and found that they are multiple epigenetic modifications in the regulation of embryonic distinct from H3K4me3 and H3K27me3 (Supplementary Fig.
Details
-
File Typepdf
-
Upload Time-
-
Content LanguagesEnglish
-
Upload UserAnonymous/Not logged-in
-
File Pages23 Page
-
File Size-