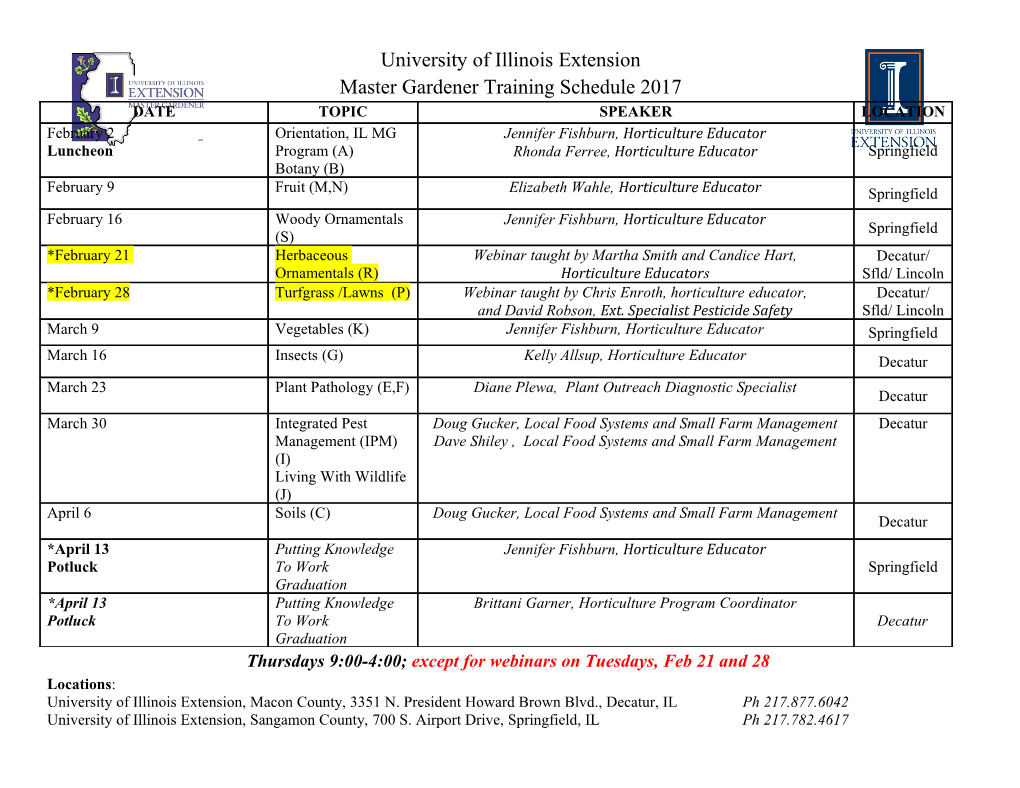
We are IntechOpen, the world’s leading publisher of Open Access books Built by scientists, for scientists 5,300 131,000 155M Open access books available International authors and editors Downloads Our authors are among the 154 TOP 1% 12.2% Countries delivered to most cited scientists Contributors from top 500 universities Selection of our books indexed in the Book Citation Index in Web of Science™ Core Collection (BKCI) Interested in publishing with us? Contact [email protected] Numbers displayed above are based on latest data collected. For more information visit www.intechopen.com Chapter 10 Fourier Transform Hyperspectral Imaging for Cultural Heritage Massimo Zucco, Marco Pisani and Tiziana Cavaleri Massimo Zucco, Marco Pisani and Tiziana Cavaleri Additional information is available at the end of the chapter Additional information is available at the end of the chapter http://dx.doi.org/10.5772/66107 Abstract Hyperspectral imaging is a technique of analysis that associates to each pixel of the image the spectral content of the radiation coming from the scene. This content can be helpful to recognize the chemical nature of the materials within the scene or to calculate their colours under particular conditions. Different solutions of hyperspectral imager have been realized with different spatial resolution, spectral resolution and range in the elec‐ tromagnetic spectrum. In particular, improving the spectral resolution allows discrimi‐ nating smaller features in the spectrum and the unambiguous detection of the absorption bands characteristic of superficial materials. Hyperspectral imagers based on interfer ‐ ometers have the advantage of having a spectral resolution that can be varied according to the needs by changing the optical path delay of the interferometer. A spectrum for each pixel is obtained with an algorithm based on the Fourier transform of the calibrated interferogram. We present the results of the application of a hyperspectral imager based on Fabry‐Perot interferometers to the field of cultural heritage. On different artworks, the hyperspectral imager has been used for pigment recognition, for colour rendering elaborations of the image with different light sources or standard illuminants and for calculating the chromatic coordinates useful for specific purposes. Keywords: hyperspectral imaging (HSI), fibre optics reflectance spectroscopy (FORS), cultural heritage, conservation, colour rendering, Fourier transform 1. Introduction Hyperspectral imaging (HSI) is a powerful technique of analysis where each pixel of the image is associated with the spectral content of the radiation coming from the scene in the spectral band of interest. Different solutions with different spectral resolutions have been © 2017 The Author(s). Licensee InTech. This chapter is distributed under the terms of the Creative Commons © 2016 The Author(s). Licensee InTech. This chapter is distributed under the terms of the Creative Commons Attribution License (http://creativecommons.org/licenses/by/3.0), which permits unrestricted use, Attribution License (http://creativecommons.org/licenses/by/3.0), which permits unrestricted use, distribution, distribution, and reproduction in any medium, provided the original work is properly cited. and reproduction in any medium, provided the original work is properly cited. 216 Fourier Transforms - High-tech Application and Current Trends adopted to separate the spectral content of the radiation impinging on the pixel: starting from the three bands of the Bayer filter camera [ 1], to the tens of bands with fixed bandpass filters like in the OSIRIS camera on Rosetta spacecraft [ 2] or hundreds or even thousands of bands of imagers based on dispersive means with gratings or prism like on the VIRTIS camera on Rosetta spacecraft [3]. In this work, we are interested in hyperspectral imagers based on inter ‐ ferometers: an interferometer is placed in the optical system in front of the camera, and while the optical path delay (OPD) of the interferometer is varied, the interferogram for each pixel is acquired by the camera and the spectrum is calculated with an algorithm based on the Fourier transform. The final attainable resolution in principle is only limited by the maximal optical path delay of the interferometer. HSI based on Michelson interferometers have been implemented with success in commercial instruments by Bruker4 ][ and Telops 5[] ensuring more than 500 bands in the infrared region and reaching a resolution of less than 1 cm−1. At INRIM, we have realized a different concept of HSI based on Fabry‐Perot interferometer (FPI) and we have validated it in different regions of the spectrum: in the UV 6[ ], in the visible [7] and in the near infrared [8] and in different applications [9]. In paragraph 2 of this chapter, we will describe the principle of the reflectance spectra calcu‐ lation based on the Fourier transform. In paragraph 3, we will show the application of this technique to the field of cultural heritage in collaboration with Centre for Conservation and Restoration La Venaria Reale (CCR). Reflectance spectra indeed contain information useful for identifying pigments and dyes and thus for discriminating original and possibly superim‐ posed materials (e.g. pictorial retouching) that is one of the main aims of a diagnostic campaign intended at preserving artworks and guiding the conservation treatment. Moreover, reflec ‐ tance spectra can be used for rendering the artworks’ colour appearance under different lights in order to choose the light source most suitable for enhancing some aesthetical aspects of the objects, for increasing the visitors’ satisfaction, in the meantime taking into account the preven‐ tive conservation principles and the standard recommendations for lighting in museums. On the other hand, the possibility of studying the pigments’ colour appearance can be of some help when choosing materials for the conservation treatment. Finally, spectra can be converted in colorimetric values for different light sources or standard illuminants useful for calculating chromatic differences for specific purposes. Results here presented concern some real artworks of different art periods (e.g. coffins from Ancient Egypt, Italian Renaissance polychrome ‐art works) and some mockups used as references made with known pigments and binders. 2. Fabry‐Perot hyperspectral imager Our hyperspectral imager (HSI) is based on a Fabry‐Perot interferometer (FPI), the optical system is represented in Figure 1 where the scene is firstly imaged in the FPI so that the trans ‐ mitted intensity is modulated by the interference, and the second image is then formed on the camera sensor by means of the relay lens. A sequence of frames carrying the interference fringe information is acquired synchronized with the scan of the optical path delay (OPD) between the mirrors of the FPI, from contact to the maximal distance of the mirror. For each pixel of the image, the interferogram is extracted from Fourier Transform Hyperspectral Imaging for Cultural Heritage 217 http://dx.doi.org/10.5772/66107 the acquired video: as an example, we present the interferogram of a light‐emitting diode (LED) at about 635 nm in Figure 2 where it is evident the non‐linearity of the actuators used to vary the OPD near the contact of the mirrors. Thex axis of the interferogram is calibrated by using a laser in the optical setup. More details of the calibration technique are described in the previous work [10]. In Figure 3, we present the calibrated, resampled and rescaled interferogram. Figure 1. The scheme of the HSI: the FPI is inserted in an optical setup and the first image of the scene is formed in the FPI, where it is modulated by the interference and the second image with the interference is formed on the camera sensor. The optical path delay is changed while the image is acquired by the camera. Figure 2. The interferogram extracted from the succession of frames of a LED at 635 nm. The x axis is the frame number. The first points of the interferogram are missing due to the penetration depth of the mirror coating. 218 Fourier Transforms - High-tech Application and Current Trends Figure 3. The calibrated, resampled and rescaled interferogram from Figure 2. The first points of the interferograms are missing due to the penetration depth of mirror coat‐ ings, and according to Fourier transform theory, they correspond to the cosine contributions having the longest period in the spectra calculation. By inserting a bandpass filter in the opti‐ cal setup and using the information that the spectrum has to be zero in certain regions of the electromagnetic spectrum, it is possible to find the amplitude of the missing points of the interferogram and reconstitute the original spectrum by applying the discrete Fourier transform (DFT) [10]. In Figure 4, we present the spectrum obtained from the DFT applied to the calibrated interferogram in Figure 3 using the Hanning apodization function. The DFT spectrum is expressed in the frequency domain, and the spectrum of interest is in the band 400–720 nm (416–750 THz) according to the bandpass filter. The peak of the LED is at about 472 THz, corresponding to 635 nm, and since the base of the FPI is the Airy function, and not the cosine as in the Michelson interferometer, DFT creates the harmonics of the peak at 472 THz that decease as Rn/n, where R is the reflectance of the mirrors andn is the order of the harmonic [11]. The interferogram in Figure 3 is obtained with a maximal OPD of about 30 µm that corresponds to a spectral resolution of about 10 THz. The frequency interval in the spec ‐ trum, as visible in the inset of Figure 4, is decreased below the spectral resolution by using the zero padding method [10]. A phase correction has been applied to the spectrum calculation in order to take into account the phase dispersion of the mirror coatings [ 11]. In the right side of spectrum are evident the aliases of the LED peak, artefacts of the DFT.
Details
-
File Typepdf
-
Upload Time-
-
Content LanguagesEnglish
-
Upload UserAnonymous/Not logged-in
-
File Pages21 Page
-
File Size-