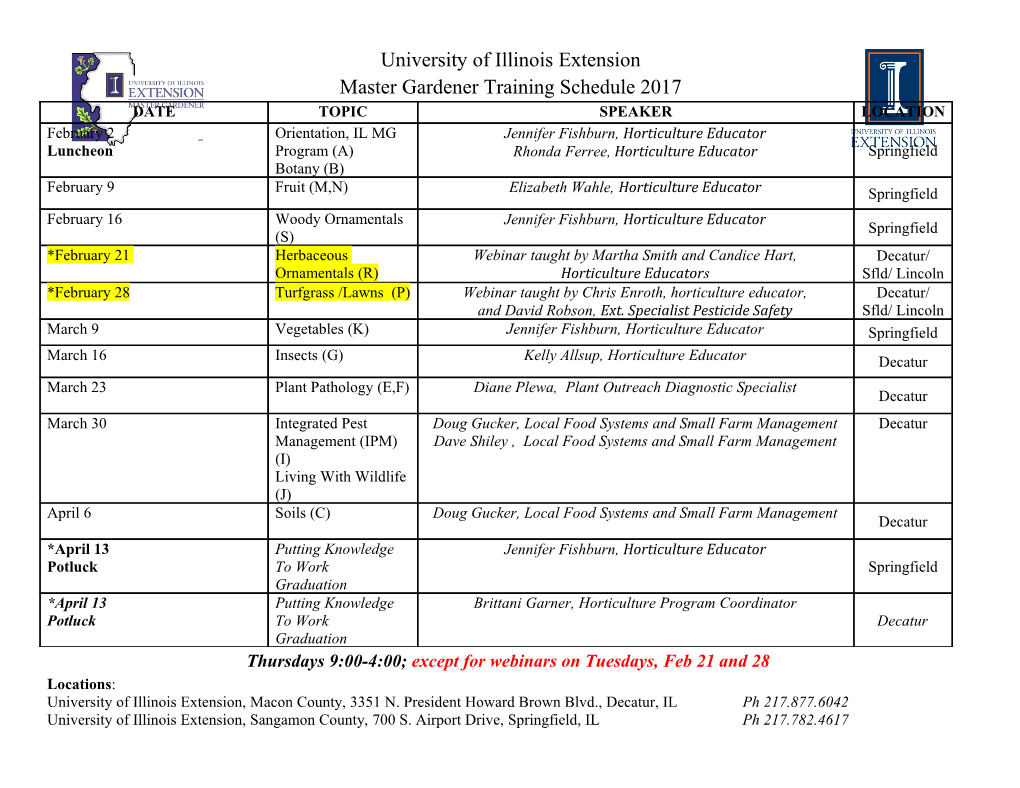
THEMED ISSUE: Tectonics at the Micro- to Macroscale: Contributions in Honor of the University of Michigan Structure-Tectonics Research Group of Ben van der Pluijm and Rob Van der Voo Structural evolution of an en echelon fold system within the Laramide foreland, central Wyoming: From early layer- parallel shortening to fault propagation and fold linkage Adolph Yonkee1 and Arlo Brandon Weil2 1DEPARTMENT OF GEOSCIENCES, WEBER STATE UNIVERSITY, 1415 EDVALSON STREET, DEPARTMENT 2507, OGDEN, UTAH 84408, USA 2DEPARTMENT OF GEOLOGY, BRYN MAWR COLLEGE, 101 NORTH MERION AVENUE, BRYN MAWR, PENNSYLVANIA 19010, USA ABSTRACT Minor fault kinematics, fracture sets, anisotropy of magnetic susceptibility (AMS) fabrics, and remanent paleomagnetism within Triassic to Jurassic red beds and limestone along the Dallas–Derby–Sheep Mountain fold system and northeast flank of the Laramide Wind River arch record patterns of progressive deformation from early layer-parallel shortening (LPS) to large-scale fault propagation and fold linkage. The fold system comprises a series of doubly plunging, left-stepping anticlines with segmented southwest-vergent forelimbs cut by reverse faults and a gently northeast-dipping backlimb. Anticlines are linked across structural saddles (relay zones) that are locally cut by steep east- striking oblique-slip faults and thrust faults that accommodated shortening transfer. Minor wedge faults within limestone show consistent relationships with respect to bedding around anticline limbs, recording early LPS prior to and synchronous with initial fold growth. Within red beds, LPS produced microkinked phyllosilicate grains that define AMS Kmax (axes of maximum susceptibility) lineations perpendicular to shortening directions. LPS directions estimated from minor faults and AMS trend approximately west-southwest–east-northeast within the backlimb, subparallel to the regional Laramide shortening direction, and partly refract across arcuate forelimbs. Paleomagnetic data record local counterclockwise rotations in forelimbs associated with map-view curvature and eastward-striking faults. Fracture sets and previously published calcite-twin strain data show more complex patterns related to evolving stress-strain fields, from pre-Laramide (intraplate) west- northwest–east-southeast compression, to early Laramide west-southwest–east-northeast LPS, to later Laramide fault propagation and local stress refraction. Reverse faults in basement rocks propagated upward into anticline forelimbs, and laterally with partial linkage by eastward- striking faults that formed along basement weaknesses. LITHOSPHERE; v. 9; no. 5; p. 828–850; GSA Data Repository Item 2017299 | Published online 9 August 2017 https://doi.org/10.1130/L622.1 INTRODUCTION 2017). In this paper structural and paleomagnetic data sets for a flanking fold system of the basement-cored Wind River arch are examined to bet- The Laramide belt, spanning a region from southern Montana to New ter understand Laramide tectonics. Note that the term Laramide is used Mexico (USA), is an archetypical example of thick-skin foreland defor- for thick-skin structural style (compared to thin-skin style of Sevier belt), mation that developed far inboard from a plate margin (Fig. 1; Dickinson and not for a specific time interval. and Snyder, 1978; Bird, 1984; Saleeby, 2003). The belt is characterized Growth of variably oriented basement-cored arches and flanking fold by fault-bound, basement-cored arches that are bordered by flanking fold systems within the Laramide belt have generated multiple tectonic mod- systems and separated by broad basins (Berg, 1962; Brown, 1988; Erslev, els, including (1) temporal changes in shortening directions (Gries, 1983; 1993). The belt displays an overall northwest-southeast structural grain, Bergh and Snoke, 1992), (2) transpression with varying components of but individual basement faults have a range of strikes and cover folds form horizontal shear along differently oriented zones (Sales, 1968; Stone, both north-trending, right-stepping, and west-trending, left-stepping en 1969), and (3) regional shortening with spatial refraction of stress-strain echelon systems. General timing of large-scale Laramide deformation directions (Fig. 2A; Erslev and Koenig, 2009). Model 1 predicts early east- spanned ca. 70–50 Ma in the Wyoming region, constrained by sedimen- west shortening that formed north-trending arches, followed by late north- tologic patterns in the basins (Beck et al., 1988; Dickinson et al., 1988; south shortening that formed east-trending arches, with overprinting and DeCelles et al., 1991; Steidtmann and Middleton, 1991; Hoy and Ridg- possible linkage of early structures by later structures. Model 2 predicts way, 1997) and by thermochronologic data in the arches (Crowley et al., widespread development of en echelon folds, strike-slip to oblique-slip 2002; Peyton et al., 2012; Stevens et al., 2016). However, patterns vary faulting, and significant vertical-axis rotations, with sinistral shear and regionally and questions remain on timing of Laramide initiation, varying left-stepping folds along more west-trending zones, compared to dextral models of southwest to northeast, west to east, or northeast to southwest shear and right-stepping folds along more north-trending zones. Model propagation of deformation, and changes in shortening directions over 3 predicts smoothly varying stress-strain directions during temporally time (Chapin and Cather, 1983; Gries, 1983; Varga, 1993; Heller et al., overlapping development of variably trending arches, partly related to 2013; Fan and Carrapa, 2014; Lopez and Steel, 2015; Copeland et al., preexisting basement heterogeneities and weaknesses (Huntoon, 1993; 828 © 2017 Geological Society of America.www.gsapubs.org For permission | toVolume copy, contact9 | Number [email protected] 5 | LITHOSPHERE Downloaded from http://pubs.geoscienceworld.org/gsa/lithosphere/article-pdf/9/5/828/3712266/828.pdf by guest on 07 October 2021 Structural evolution of an en echelon fold system | THEMED ISSUE A Paleogene ~ 60-50 Ma Current o o o 111 110 109o 108o 107o 106o 105 45o Beartooth Arch Canada Black Hills U.S. Neogene Arch Volcanics Absaroka Bighorn Powder Volcanics Arch River North Basin American Bighorn Basin o gmat Ma plate 44 Salitzia ic ar ic c X2 Owl Creek Arch s Wind accreted River Fig. 3A o terrane Basin 43 Wind River Arch vier ftb Sweetwater Si erra Nevada arc (ina s Arch Se amide belt Green Lar River Laramie Basin Arch X1 F hinterland r a Great o n 42 c core complexe t i s Rock c Moxa Hanna Basin a Valley n Arch Springs ct x S Arch u ive) xenoliths Colo Min Belt b Fo d x N u rearc x c t C x o m Wyoming Salien Medicine p M le ojave se Bow x Arch WYOMING 41o ct a or segmet-slab disrupted 050 100 km Sevier thrust Eocene volcanics Farallon n for t Laramide basins plate ear Laramide reverse fault c (dashed - concealed) with axis Anticlinal fold trace Triassic red beds normal fault Precambrian basement Paleogene ~ 60-50 Ma B hinterland plateau X1 Sevier Laramide X2 Subduct Inactive arc core complexes fold-thrust belt foreland belt complex Forearc Accreted sed cover terranes basement Farallon oceanic mantle plate lithosphere end loading n lithosphericric keelkeeel increased relative plate motio [NE-underthrust at slab] basal traction basal drag plateauteau cruscrc t ~100 km astheno- sphere ow Figure 1. (A) Generalized tectonic map of the Cordilleran system (left) illustrates setting of Laramide foreland belt and Sevier fold-thrust belt during the Paleogene; interpreted extent of flat-slab segment is indicated. Generalized geologic map of Wyoming (right) shows Laramide major fault traces, fold traces, basin axes, and outcrop distributions of Precambrian basement and Triassic red beds, and major thrust traces of the Sevier belt. Box out- lines general study region shown in Figure 3A. (B) Schematic tectonic cross-section X1–X2 across the Cordilleran system shows development of the Laramide foreland belt during flat-slab subduction related to possible increases in end loading, basal traction, and asthenospheric flow. Modified from Saleeby (2003) and Yonkee and Weil (2015). Molzer and Erslev, 1995; Marshak et al., 2000; Stone, 2002; Neely and Relations of flanking fold system formation to basement deformation Erslev, 2009; Weil et al., 2014). For this model, along-strike fault propa- have also generated multiple models, including (i) detachment and buckle gation and fold linkage may also give rise to either left- or right-stepping folding of the sedimentary cover related to crowding (Petersen, 1983; Nel- en echelon fold systems, along with local partitioning of shear along son, 1993); (ii) fault-propagation folding associated with basement reverse obliquely oriented faults. It is important that these models predict differ- faults, as in fold-thrust and trishear models (Berg, 1962; Erslev, 1991; ent spatial and temporal patterns of large-scale fault and fold geometry, Stone, 1993); and (iii) drape folding above subvertical basement faults internal strain, and vertical-axis rotations, which can be evaluated from (Fig. 2B; Matthews and Work, 1978). For detachment models (i), folds integrated structural and paleomagnetic studies. Strike tests, in which should be broadly symmetric and associated with listric thrust faults. For changes in shortening directions and paleomagnetic declinations are cor- fault-propagation models (ii), fold forelimbs should be steeper and undergo related with changes in structural trend, provide a quantitative
Details
-
File Typepdf
-
Upload Time-
-
Content LanguagesEnglish
-
Upload UserAnonymous/Not logged-in
-
File Pages23 Page
-
File Size-