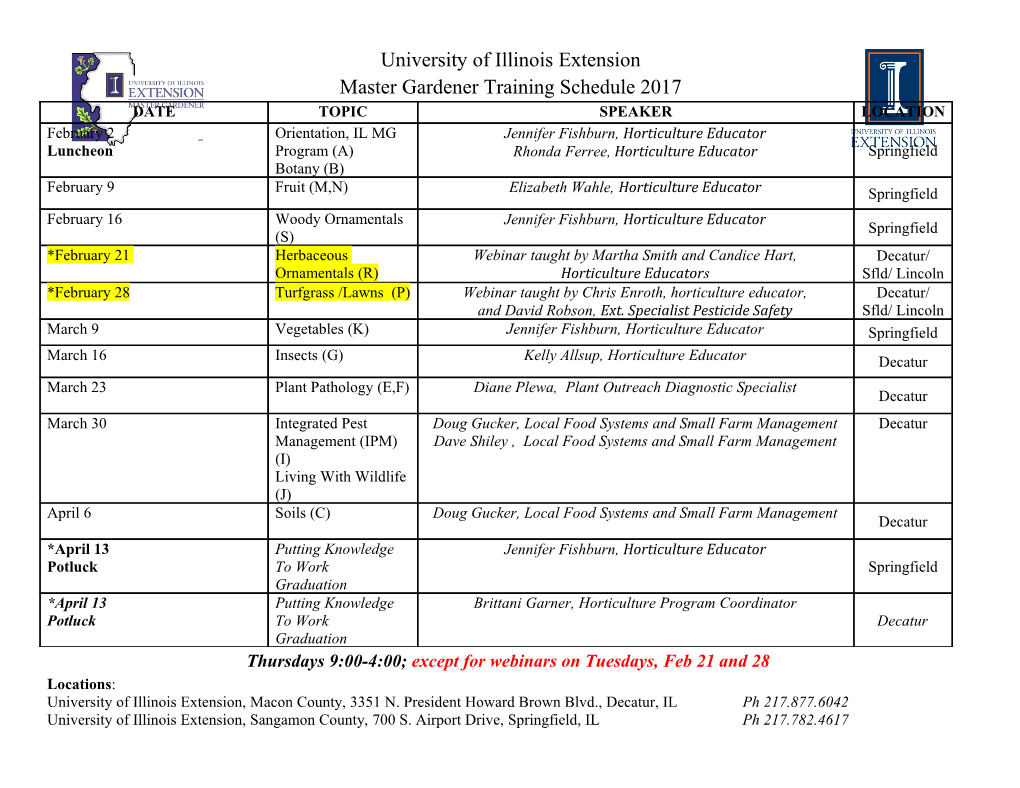
Principles of Raman Spectroscopy Salvatore Amoruso Lectures for the course of Atomic and Molecular Physics and Laser Spectroscopy 1 Raman scattering Raman Spectroscopy is based on Raman scattering Raman effect was first observed in 1928 and was used to investigate the vibrational states of many molecules in the 1930s. Raman discovered that a very small fraction of the scattered light was present at wavelength different from the incident one (Raman shift), and got the Nobel prize in 1931. Raman Inelastic scattering Initially, spectroscopic methods based on the phenomenon were used in research on the structure of relatively simple molecules. However, the development of laser sources and new generations of monochromators and detectors has made possible the application of Raman spectroscopy to the solution of many problems of scientific and technological interest. Scattering We already studied the Rayleigh (elastic scattering) at the very beginning of the course. We discussed it as due to the re-emission of light from the molecular dipole induced by the incoming beam! Linear medium Pt 0 Et Molecule induced electronic dipole pt q r t E expik r t e e 0 0 pt E 0 exp it From the Lorentz model we got Rayleigh (elastic) scattering formula 1 I 4 s 4 Raman Scattering: classical description Consider now molecular vibrations at v pt qere t r0 cosvt v Vibrating molecular dipole v r rr0 To make the formula easier cos t cos t 0 r v v 0 v v pt Et cos tE cost 0 v v 0 E E E cost v 0 cos t v 0 cos t 0 0 2 v 2 v Raman Scattering: classical description 2 2 4 2 2 4 2 2 4 2 E E E I p 0 0 v 0 v v 0 v s 2 8 8 Rayleigh Raman Stokes Anti-Stokes Typically Raman scattering << Rayleigh scattering -5 -6 -2 -3 Is(10 -10 ) Iinc Is (10 -10 ) Iinc v r rr0 Raman scattering depends on induced changes of polarizability, i.e. on the oscillation of the electronic charges in response to the incident field. Raman Scattering: classical description Very weak signal Linear response (Is,RIinc) Characteristic signature of the molecule vibrations Laser Raman Spectroscopy Stokes vs anti-Stokes 4 Is Stokes v 4 1 >> v) Is anti Stokes v Experimentally Is(Stokes) > Is(anti-Stokes) Raman effect: quanto-mechanical description Virtual states IR absorption Raman effect: quanto-mechanical description Molecule: electronic levels + vibrational levels Interaction with the e.m. field As for classical Raman scattering depends on induced polarizability, i.e. on the oscillation of the electronic charges in response to the incident field. Stokes anti-Stokes N(n=1)=N exp(-hn /k T) N(n=0)=N0 0 v B Is(Raman) N(n) 4 Is Stokes n n v hn v 4 exp >> 1 Is anti Stokes n n v kBT At high temperature of the sample the ratio can be used to measure the temperature 4 Is Stokes n n v hn v 4 exp Is anti Stokes n n v kBT Example of a Raman spectrum: carbon tetracloride CCl4 Example of a Raman spectrum: Benzene 992 cm-1 3062 cm-1 3046 cm-1 -1 -1 Intensity 1595 cm 1178 cm 606 cm-1 849 cm-1 Raman-shift [cm-1] Raman vs IR spectroscopy Both involve vibrational levels IR: absorption of radiation Raman: scattering of radiation Some lines present in the IR spectrum are not present in Raman and viceversa Vibrational Modes Raman-shift = frequency of the normal vibration mode 3N-6 normal vibration modes a a Induced polizability Qk Qk Qk ,0 Raman vs IR spectroscopy Selection rules Raman Gross selection rule - The polarizability a must change during the vibration a a a a Q Qk 0 k 0 Qk Q 0 Qk k Qk Qk ,0 IR Gross selection rule - The molecule dipole momentum must change during the vibration Q Qk 0 k 0 Qk Q 0 k Qk Qk Qk ,0 Transition probability Raman a a 1 T Q Q Q nn' n' n Q n' n Q Q Q0 Q Q0 2 IR 1 T Q Q Q nn' n' n Q n' n Q QQ0 QQ0 2 Specific selection rule Gross selection rule for harmonic oscillator related to variation of dipole Dn=1 moment or polarizability Example: linear molecule CO2 0 a changes Linear, symmetric molecule IR Raman active Example: CO2 The rule of mutual exclusion It si not a pure coincidence that in the case of CO2 Raman active modes are IR inactive and vice versa. This is an example of the rule of mutual exclusion. In centrosymmetric molecules (i.e. those with a center of inversion symmetry) a vibrational mode may be either IR active or Raman active, but not both. Another example: symmetric and asymettric biatomic molecules e.g. H2, N2, O2 e.g. CO Raman active modes Raman active Another example: symmetric and asymettric biatomic molecules e.g. H2, N2, O2 e.g. CO IR active modes IR active Other molecules For polyatomic molecules or asymmetric molecules there is need to check the Gross Selection Rule for any normal mode of vibration THIS CAN BE VERY COMPLEX . … and one should refer to the existing literature. IR and Raman offers complementary techniques Raman IR Weak signal intensity High background (absorption) Low background (scattering) More bands . (once Rayleigh component is removed) . but Complex spectra Low Raman signal of water and glass (important for biological Infrared sources and detectors samples, etc..) Dn shift independent of inc, the Characteristic signature of lines are narrower species Possibility to work in UV-Vis- Near IR region Applicable to gas, liquid and (good sources and detectors) solid samples Characteristic signature of species Can also access range not available for IR (e.g. 100-700 cm-1) Lattice vibrations It’s a salt RAMAN SPECTROMETER filter Spectral light source selection and recording sample Removal of Rayleigh scattering can be accomplished by approprieate filters or spectral selection apparatus (double or triple spectrograph) Usually Sokes emission is recorded Notch filter @ 633 nm Intense monochromatic light source provides higher signal and narrow lines LASER Which excitation wavelength is worth using ? Argon laser: 514 nm and 488 nm Nd:Yag laser: 1064 nm, 527 nm, 355 nm, 263 nm He:Ne: 632.5 nm Diode lasers: 976, 830, 780, . , . nm 1 I 4 s v 4 UV better than VIS better than IR 263 nm vs 1064 nm Gain a factor 256 BUT 527 nm vs 1064 nm Gain a factor 16 BUT Drawback Fluorescence Fluorescence can hinder the Raman signal or complicate the analysis Molecular damage Absorption can lead to damage of the sample Opportunity Resonant Raman Raman scattering excited close to a real state can enhance the signal by order of magnitude Fluorescence Raman spectra of a dye molecule Scattering is simultaneous to excitation Fluorescence occurs on longer time scale Appropriate gating could allow separating the two signals Another example Poly (9 vinylcarbazol) is a polymer used for OLED fabrication Resonant Raman Higher sensitivity (enhancement up to 106) absorption Raman Resonant Raman Resonant Raman can be applied to excite specific parts of big molecules (as for instance in biological samples) used as cromophores or markers Resonant Raman vs Raman spectrum Para-ethyl phenols (PEP) in hexane (solvent) | PEP - * solvent Appropriate selection of the excitation wavelength enhances the signal of the PEP only Clearly seen in three spectral regions without solvent interference Para-ethyl phenol Present in beer and wine Resonant Raman of nanostructures – GaAs/AlAs Superlattices Resonant Raman of nanostructures – ZnSe nanowires EXPERIMENTAL FACTS SCATTERING PROBABILITIES AND GEOMETRY 3 P 1 cos2 Rayleigh 4 3 2 PRaman 1 3 1 cos 4 8 is the depolarization ratio. For =0, same dependence P 0,180 2 P 90 Backscattering and transmission can allow gaining up to factor 2 in the probability of the scattering. The actual intensity ratio depends on the molecule characteristics (e.g. . Example: N2 molecule (=0.19 @=337.1 nm I 180 Raman 5104 IRayleigh 180 Other considerations Backscattering P 180 Raman 1.50 - need for access to one sample surface only, PRaman 90 useful for solids, thin films, etc.. - higher Raman scattering efficiency 90° collection PRaman 90 PRaman 180 1.34 Raman/Rayleigh ratio more favorable PRayleigh 90 PRayleigh 180 The scattered light is collected by a large condensing lens and sent to a monochromator and detector, typically an array or CCD Problem: we need to remove Rayleigh component and get high resolution BIG Raman Systems: double and triple monochromators Double monochromator Typical focal length 1 m Triple monochromator Intensity variation of scattered ligth for single, double and triple grating spectrometers. COMPACT Raman System: Notch filters and small monochromators Notch filter @ 633 nm Filters must be changed according to the excitation laser wavelength Analysis of pharmaceutical products transmission vs traditional Raman spectra SAMPLES ONE APPLICATION TO ATMOSPHERIC SOUNDING THE RAMAN LIDAR (use intense pulsed laser sources) NOT ENOUGH TIME FOR MANY DETAILS JUST PRINCIPLES OF THE TECHNIQUE Backscattered signals due to elastic and anelastic (Raman) scattering Elastic (Mie, Rayleigh) (molecules + aerosol) @ exc = 527 nm Anelastic (Raman) only specific molecules Raman,N2= 627 nm FROM Raman backscatter spectrum of the atmopsphere inc=355 nm Schematic of a Raman lidar for H2O mixing ratio measurements H2O mixing ratio measurements: an example RAMAN MICROSCOPY Sketch of a Raman Microscope Confocal Normal SOME EXAMPLES OF APPLICATIONS – Qualitative analysis Example 1 - material crystalline phase identification: TiO2 Example 2 – modification induced by treatment Laser microfabrication Target scanning 300 fs pulses at 527 nm 300 fs pulses at 263 nm Nanosecond pulses induce damage and modification of the sample.
Details
-
File Typepdf
-
Upload Time-
-
Content LanguagesEnglish
-
Upload UserAnonymous/Not logged-in
-
File Pages68 Page
-
File Size-