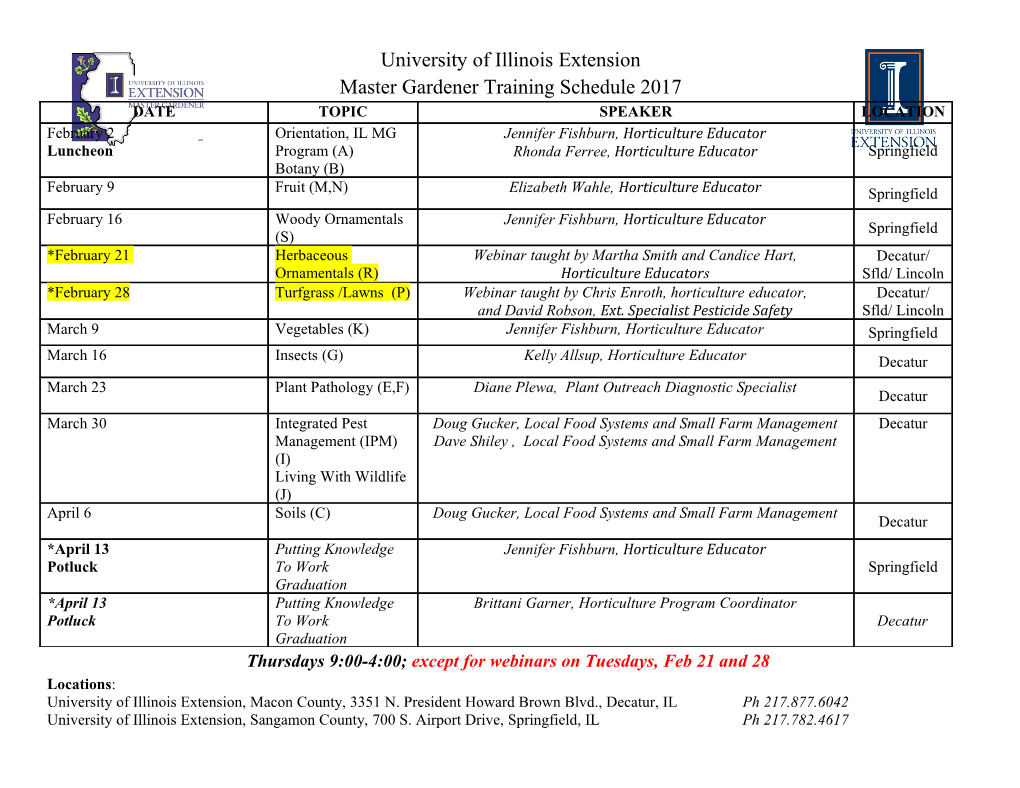
Proc. Natl. Acad. Sci. USA Vol. 93, pp. 5443-5448, May 1996 Biochemistry Conserved motifs in prokaryotic and eukaryotic polypeptide release factors: tRNA-protein mimicry hypothesis (translation termination/stop codon recognition/elongation factor G/molecular mimicry) KoICHI ITO, KANAE EBIHARA, MAKIKO UNO, AND YOSHIKAzu NAKAMURA* Department of Tumor Biology, Institute of Medical Science, University of Tokyo, 4-6-1 Shirokanedai, Minato-ku, Tokyo 108, Japan Communicated by Charles Yanofsky, Stanford University, Stanford, CA, January 31, 1996 (received for review November 1, 1995) ABSTRACT Translation termination requires two codon- domain motifs and is involved in omnipotent suppression of specific polypeptide release factors in prokaryotes and one nonsense codons (for a review, see ref. 11). omnipotent factor in eukaryotes. Sequences of 17 different Can the current computer programs used for sequence polypeptide release factors from prokaryotes and eukaryotes comparison, as designed, predict conserved amino acids at were compared. The prokaryotic release factors share resi- discrete positions in comparisons of multiple random se- dues split into seven motifs. Conservation of many discrete, quences? It seems unlikely to us that the currently used perhaps critical, amino acids is observed in eukaryotic release computer programs would recognize single conserved amino factors, as well as in the C-terminal portion of elongation acids when the number and diversity of protein sequences is factor (EF) G. Given that the C-terminal domains of EF-G increased, because the algorithms used are essentially based on interacts with ribosomes by mimicry of a tRNA structure, the one-to-one comparison of letters or words of finite length. pattern of conservation of residues in release factors may Here, we approach this problem by identifying first "by reflect requirements for a tRNA-mimicry for binding to the A computer" the conserved amino acids in prokaryotic RFs, and site of the ribosome. This mimicry would explain why release then asking "by eye" whether these residues are also present factors recognize stop codons and suggests that all prokary- in eukaryotic RFs. This approach provided us with clues that otic and eukaryotic release factors evolved from the progen- lead to universally conserved motifs in RFs, part of which may itor of EF-G. reflect requirements for molecular mimicry of a tRNA struc- ture. The termination of translation in bacteria requires two codon- specific peptide chain release factors (RF): release factor-1 MATERIALS AND METHODS (RF-1; UAG/UAA specific) and release factor-2 (RF-2; UGA/UAA specific) (1). A third factor, release factor-3 Sup45 Gene of Schizosaccharomyces pombe. The Sup45 gene (RF-3), is known to stimulate the activities of RF-1 and RF-2 of the fission yeast Sch. pombe was cloned by DNA hybrid- and to bind guanine nucleotides, but is not codon-specific ization using the S. cerevisiae SUP45 probe. The size of the (2-6). The mechanism of stop codon recognition by RFs EcoRI fragment encoding S. pombe Sup45 was estimated to be is unknown and "10 kb by Southern blot hybridization using the S. cerevisiae is of considerable interest since it entails SUP45 probe isolated from pUKC802 (gift from M. F. Tuite, protein-RNA recognition rather than the well-understood University of Kent; see ref. 13). A shotgun library of Sch. mRNA-tRNA interaction in codon-anticodon pairing (7, 8). pombe EcoRI DNAs in ADASH II vector (Stratagene) was The existence of a protein with RF activity in eukaryotes was plaque-screened. After several rounds of screening, positive demonstrated some 20 years ago in rabbit reticulocytes (9). phage clones were characterized by genetic and DNA sequenc- After two decades of investigation, a eukaryotic protein family ing analyses. The 6-kb Sall-Sall fragment subcloned in plasmid with the properties of RFs, designated eRF-1, has been pRS316 (14) encoded a Sup45 homolog which is able to discovered (10). Two members of the eRF-1 family, one from complement intergenerically the S. cerevisiae sup45 mutation. humans and the other from Xenopus laevis, have been purified Streptomycetes RF-2 Gene. Streptomycetes are mycelial, and shown to catalyze polypeptide release at all three stop Gram-positive bacteria that produce a wide variety of antibi- codons in vitro. Another protein in this family, Sup45 of the otics and bioactive compounds. The RF-2 gene ofStreptomyces budding yeast Saccharomyces cerevisiae, is involved in omnip- coelicolor A3(2) was found in a 6.3-kb DNA fragment also otent suppression during translation (for a review, see ref. 11). encoding protein kinases (15). DNA sequence analysis re- However, the discoverers of the eRF-1 family failed to detect vealed that Streptomyces RF-2 comprises 368 amino acids and any sequence resemblance between bacterial RFs (including a is 43-45% identical to Escherichia coli, Salmonella typhi- yeast mitochondrial RF, mRF-1) and eukaryotic RFs, and murium, or Bacillus subtilis RF-2. The Streptomyces RF-2 gene speculated that the proteins responsible for eukaryotic termi- was able to complement the E. coli prfB mutations (15). nation evolved independently from the RFs of prokaryotes Dominant Lethal Mutations in E. coli RF-2 Gene. Plasmid (10). pSUIQ-RF2+ is an isopropyl ,B-D-thiogalactoside (IPTG)- It appeared unlikely that eRF-1 is the only RF required for controllableprfB+ expression plasmid that contains the entire polypeptide termination in eukaryotes because no member of p+B' encoding sequence ofE. coli under the lac promoter and the eRF-1 family contained a consensus G-domain structure. the lacIq sequence. The pSUIQ-RF2+ complements the E. coli A GTP requirement for polypeptide release was demonstrated some 20 years ago in rabbit reticulocytes (9). Eukaryotic Abbreviations: RF, release factor; RF-1, release factor 1; RF-2, release counterparts of bacterial RF-3 have been proposed recently factor 2; RF-3, release factor 3; eRF-1, eukaryotic release factor 1; and referred to as eRF-3 (12). The reported eRF-3 family eRF-3, eukaryotic release factor 3; mRF-1, mitochondrial release includes a Sup35 protein of S. cerevisiae, which carries G- factor 1; IPTG, isopropyl P3-D-thiogalactoside; EF, elongation factor. Data deposition: The sequences reported in this paper have been deposited in the GenBank data base (accession nos. D63883 and The publication costs of this article were defrayed in part by page charge D26540). payment. This article must therefore be hereby marked "advertisement" in *To whom reprint requests should be addressed. e-mail: nak@hgc. accordance with 18 U.S.C. §1734 solely to indicate this fact. ims.u-tokyo.ac.jp. 5443 Downloaded by guest on September 25, 2021 5444 Biochemistry: Ito et al. Proc. Natl. Acad. Sci. USA 93 (1996) prfB mutations in the presence of IPTG. The derivatives Five eRF-1 sequences from human, Xenopus laevis, Arabi- carrying the prfB2070 and prfB2130 mutations were con- doposis thaliana, S. cerevisiae (10), and Sch. pombe (this study) structed by creating single amino acid substitutions in pSUIQ- were aligned first by using the BESTFIT or PILEUP programs. RF2+ DNA at positions 207 and 213 by site-directed mu- Then, we compared "by eye" the yeast Sup45 sequences with tagenesis (16) by using primers 5'-AAAGCCCGACT- the prokaryotic alignment by assuming appropriate, but min- GACTCCG-3' and 5'-GCGGTCGTATCCACACGT-3', imal, gaps to achieve an optimal match. Residues that were respectively (base substitutions are underlined). Details of identical or at which there were conservative replacements in these and other plasmid constructions will be published else- comparisons with the yeast Sup45 sequences are shown in Fig. where. 2. Seven prokaryotic RFs and four eRF-ls that are distantly related are compared. Most of the amino acids from the eukaryote proteins assigned using this strategy coincided with RESULTS AND DISCUSSION those highly conserved among the prokaryotic RF sequences, S. pombe Sup45 Gene Structure. Two novel RF genes that suggesting that there is a pattern of conservation of many are from species distantly related to those with known RF discrete, perhaps critical, amino acids in all RFs. One third of genes were cloned and sequenced to facilitate the sequence the amino acid positions of RFs were assigned to those having comparisons. One encoded the Sup45 homolog of the fission identical residues in both prokaryotic and eukaryotic RFs, and Sch. and the other encoded an RF-2 of half of them were assigned to those having similar residues. In yeast, pombe, Strep- contrast, amino acids located at positions between these tomyces coelicolor (15). The sup45 gene of Sch. pombe encodes conserved residues are divergent between prokaryotic RFs a polypeptide of 434 amino acids and is homologous to other and eRF-ls. eRF-1 genes. It shares 63% amino acid identity to S. cerevisiae It is perhaps highly relevant that the four gaps identified in Sup45 and complements the S. cerevisiae sup45 mutation. The comparisons with Sup45 (eRF-1) exactly coincide with the S. cerevisiae strain MT557/ld (sal4-2 ura3-1 ade2-1 leu2-3, 112 junctions between conserved prokaryotic regions, A-B, B-C, MATa) cannot grow at 37°C owing to a temperature-sensitive D-E, and E-F (see Fig. 2). The gap between regions D-E allele, sal4-2, in the SUP45 gene (17). MT557/ld cells trans- corresponds to a papain-sensitive cleavage site in bacterial RFs formed at 25°C with a pRS316 derivative carrying the cloned (27), perhaps reflecting the boundary of predicted domains in Sup45 homolog of Sch. pombe became viable at 37°C, showing protein conformation. Moreover, all 14 of the RF mutations intergeneric complementation of S. cerevisiae sup45 with the whose amino acid substitutions have been determined (17, Sch. pombe counterpart. 28-33) affect the conservative amino acids or neighboring Conserved Motifs in Prokaryotic and Eukaryotic RFs.
Details
-
File Typepdf
-
Upload Time-
-
Content LanguagesEnglish
-
Upload UserAnonymous/Not logged-in
-
File Pages6 Page
-
File Size-