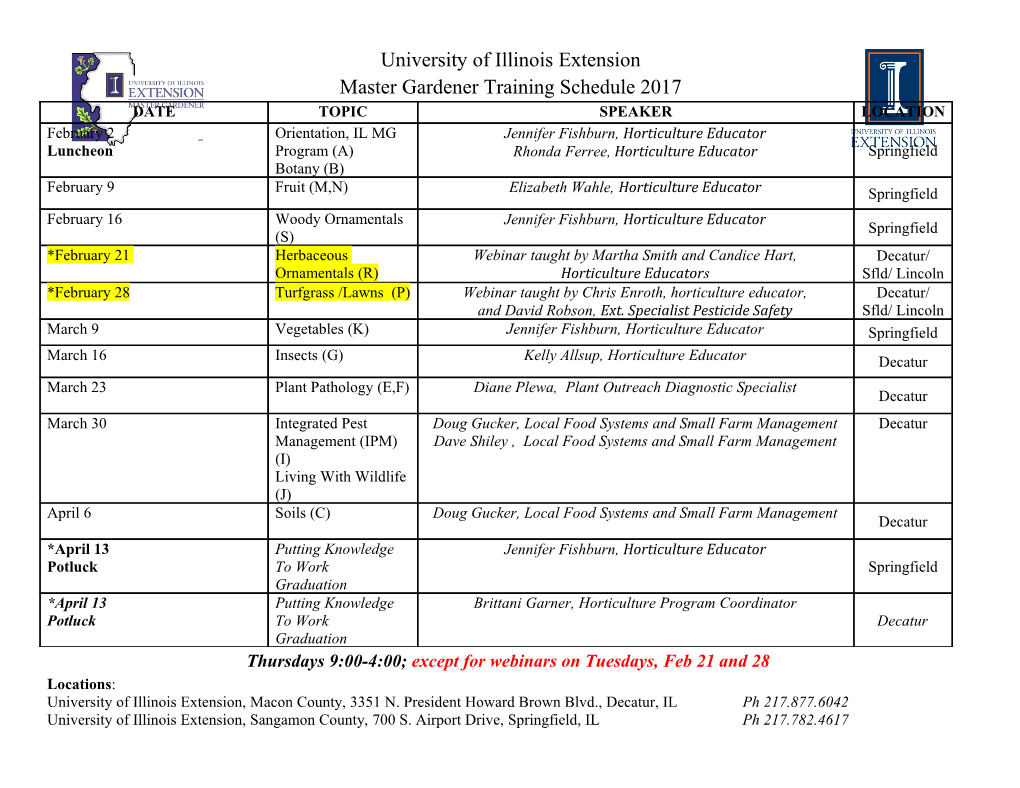
International Journal of Environmental Research and Public Health Article Screening the Capacity of 34 Wetland Plant Species to Remove Heavy Metals from Water Maria Schück * and Maria Greger Department of Ecology, Environment and Plant Sciences, Stockholm University, 106 91 Stockholm, Sweden; [email protected] * Correspondence: [email protected] Received: 27 May 2020; Accepted: 22 June 2020; Published: 27 June 2020 Abstract: Floating treatment wetlands (FTWs), consisting of vegetated rafts, may reduce heavy metal levels in polluted water, but the choice of plant species for efficient metal removal needs to be further investigated. We screened the capacity of 34 wetland plant species to remove metals dissolved in water to identify suitable species for FTWs. The plants were grown hydroponically for 5 days in 1 1 1 1 a solution containing 1.2 µg Cd L− , 68.5 µg Cu L− , 78.4 µg Pb L− , and 559 µg Zn L− . Results show large variation in metal removal rate and capacity between the investigated species. The species with highest removal capacity could remove up to 52–94% of the metals already after 0.5 h of exposure and up to 98–100% of the metals after 5 days of exposure. Plant size contributed more to high removal capacity than did removal per unit of fine roots. Carex pseudocyperus and C. riparia were the most efficient and versatile species. The findings of this study should be considered as a starting point for further investigation of plant selection for improved water purification by FTWs. Keywords: heavy metal removal; hydroponic; phytoremediation; wetland plants; water purification 1. Introduction Wetland plant species are able to remove heavy metals from the surrounding environment, which can be utilized for purification of polluted waters. One such application is treatment of polluted stormwater, which is a growing problem due to increased traffic and heavy rains [1]. A common management of stormwater is stormwater ponds that collects and delays large water masses to prevent flooding. In these ponds, particulate heavy metals are effectively removed through sedimentation, but dissolved metals, proven to be more bioavailable than particulate metals, are insufficiently removed [2,3]. Heavy metals Cd, Cu, Pb, and Zn are the most problematic heavy metals in stormwater, since they are abundant, toxic, and risk to exceed threshold values in the outlet water [4]. The use of floating treatment wetlands (FTWs) has been proposed to increase the metal removal efficacy of stormwater ponds [5,6]. FTWs consist of rafts supporting emergent plants growing hydroponically, providing direct contact between the plant roots and the polluted water [7]. Compared with conventional constructed wetlands, FTWs require no additional land use because they can be placed in existing ponds. Moreover, FTWs tolerate large variation in water level, a common problem for plants in conventional wetlands. A few mesocosm and field studies of metal removal by FTWs have been conducted, most finding decreased concentration of both dissolved and particulate metals in the outlet water [2,6,8,9], in addition to increased metal concentration in the sediment [6,10] and in the plants [11–13]. Plants are necessary for the removal of metals by FTWs [2]. The root mass reduces the water velocity, allowing small particulate metals to settle out. Root activity and the microbial processes of root-living organisms may also change the chemical environment (e.g., pH and oxygenation), increasing the sedimentation or adsorption of metals [7]. Moreover, metals follow the water uptake Int. J. Environ. Res. Public Health 2020, 17, 4623; doi:10.3390/ijerph17134623 www.mdpi.com/journal/ijerph Int. J. Environ. Res. Public Health 2020, 17, 4623 2 of 12 into the plant, further decreasing the metal content in the water. Root activity and water uptake and, consequently, removal efficiency increase with increasing root biomass [14]. Various metals are taken up to different extents by plants. Zinc is generally accumulated to a higher extent than is Cu, followed by Cd and Pb [15,16]. These differences likely depend both on the selective uptake mechanisms of plants and on the availability of metals in water due to differences in retention time. In stormwater, the dissolved fractions easily available for uptake were found to be 5%, 38%, 53%, and 59% for Pb, Cu, Zn, and Cd, respectively [3]. The ability to accumulate metals is not the same in all species. Studies have found that species differ in their removal capacity due to differences in their uptake per unit biomass [17] or differences in plant size [16], resulting in differences in total removal per plant [12,18] or per vegetated area [2]. Furthermore, studies of plants in biofiltration systems have shown that bioaccumulation differs between families [15] and clades [19]. There is clearly potential to increase the efficacy of metal removal by FTW through identifying efficient plant species or families. Most studies of metal uptake, however, consider only one or a few species and one or a few metals. To our knowledge, no study has compared the ability of many plant species to remove several metals under identical conditions. Nevertheless, screening the performance of plants by exposing them to the same conditions allows an easy comparison between species [20]. Using a hydroponic setup for the comparison has been demonstrated as a useful approach to compare and identify species that performs well in field [21]. This study aims to identify species suitable for removing Cd, Cu, Zn, and Pb in FTWs by comparing 34 wetland plant species by quantifying the removal capacities and removal rates for these metals. The metals chosen represent abundant problematic heavy metals in stormwater, a potential target for phytoremediation with the investigated species. 2. Materials and Methods 2.1. Plant Materials Specimens of 34 wetland plant species found in Swedish nature were used in the experiment (Table1). They were either collected in the wild in the Stockholm area of Sweden, grown from seed in a climate chamber, or purchased from a seller of wild plants propagated from seeds collected in Sweden (Veg-Tech AB). The species naturally grow in freshwater, brackish water, or both (Table S1). Species that grow into large plants in the wild were generally represented in this study by large specimens; similarly, small-growing species were represented by small specimens. Table 1. Biomass and origin of species included in the experiment. n = 3, SE. ± Biomass Dry Weight (g) Origin of Coarse Plant Type, Family, Species Stalk, Fine Roots Roots, Leaves Total Material a Inflorescence Rhizomes Fern, Dryopteridaceae Dryopteris carthusiana 0.71 0.17 9.22 0.81 3.13 0.64 -- 13.06 0.19 5 ± ± ± ± Monocot, Butomaceae Butomus umbellatus 0.08 0.01 0.74 0.21 1.16 0.27 - - 1.98 0.15 1 ± ± ± ± Cyperaceae Bolboschoenus maritimus 0.80 0.32 1.47 0.69 0.88 0.26 0.74 0.26 3.88 0.97 2 ± ± ± ± ± Carex acuta 0.22 0.05 0.21 0.15 0.47 0.15 - - 0.89 0.25 3 ± ± ± ± Carex cansescens 0.05 0.01 0.02 0.01 0.19 0.05 - - 0.26 0.06 3 ± ± ± ± Carex elata 0.37 0.04 0.14 0.03 1.24 0.19 - - 1.74 0.23 1 ± ± ± ± Carex flava 0.17 0.03 0.10 0.01 0.58 0.07 0.14 0.01 0.91 0.05 4 ± ± ± ± ± Carex panicea 0.18 0.02 0.13 0.02 1.05 0.15 - - 1.36 0.18 4 ± ± ± ± Carex paniculata 0.51 0.06 0.58 0.15 4.31 0.99 - - 5.41 0.16 1 ± ± ± ± Carex pseudocyperus 1.16 0.21 0.18 0.08 3.36 0.12 - - 4.71 1.32 5 ± ± ± ± Carex riparia 0.44 0.03 0.29 0.05 4.51 0.15 - - 5.23 0.15 3 ± ± ± ± Int. J. Environ. Res. Public Health 2020, 17, 4623 3 of 12 Table 1. Cont. Biomass Dry Weight (g) Origin of Coarse Plant Type, Family, Species Stalk, Fine Roots Roots, Leaves Total Material a Inflorescence Rhizomes Carex rostrata 0.64 0.23 0.40 0.13 1.77 0.66 0.26 0.00 2.86 0.57 5 ± ± ± ± ± Carex vesicaria 0.46 0.11 0.23 0.04 1.86 0.12 - - 2.55 0.16 4 ± ± ± ± Eleocharis mamillata 0.15 0.12 0.04 0.02 - - 0.67 0.02 0.86 0.84 5 ± ± ± ± Eriophorum angustifolium 0.10 0.02 0.06 0.03 0.18 0.04 - - 0.34 0.04 6 ± ± ± ± Schoenoplectus 0.40 0.01 0.51 0.18 - - 2.05 0.36 2.96 0.16 2 tabernaemontani ± ± ± ± Scirpus sylvaticus 0.43 0.04 0.29 0.05 1.51 0.16 - - 2.23 0.22 5 ± ± ± ± Iridaceae Iris pseudocourus 0.97 0.09 2.41 0.69 2.64 0.19 - - 6.03 0.80 5 ± ± ± ± Juncaceae Juncus effusus 0.58 0.01 0.27 0.02 2.42 0.14 - - 3.27 0.16 3 ± ± ± ± Poaceae Glyceria maxima 1.96 0.31 1.08 0.24 2.64 0.70 2.80 0.84 8.44 1.67 5 ± ± ± ± ± Leymus arenarius 0.09 0.02 0.10 0.02 0.30 0.00 0.27 0.01 0.77 0.01 2 ± ± ± ± ± Molinia caerulea 0.70 0.04 0.23 0.02 3.01 0.15 - - 3.93 0.16 1 ± ± ± ± Phalaris arundinacea 0.40 0.06 0.15 0.01 0.72 0.15 0.69 0.09 1.80 0.41 1 ± ± ± ± ± Phragmites australis 0.30 0.06 0.59 0.10 0.56 0.06 1.05 0.19 2.66 0.21 1 ± ± ± ± ± Typhaceae Typha latifolia 0.14 0.02 0.86 0.12 1.67 0.11 - - 2.67 0.06 5 ± ± ± ± Dicot, Asteraceae Eupatorium cannabinum 0.35 0.03 0.37 0.15 1.12 0.28 1.68 0.36 3.53 0.08 1 ± ± ± ± ± Tripolium pannonicum 0.46 0.08 0.50 0.07 0.43 0.12 - - 1.39 0.21 1 ± ± ± ± Lamiaceae Lycopus europaeus 0.09 0.02 0.08 0.03 0.61 0.11 0.27 0.05 1.04 0.15 5 ± ± ± ± ± Stachys palustris 0.30 0.07 0.27 0.06 0.85 0.04 1.14 0.09 2.47 0.57 1 ± ± ± ± ± Lythraceae Lythrum salicaria 0.25 0.05 0.56 0.12 0.39 0.11 1.43 0.12 3.24 0.13 1 ± ± ± ± ± Plantaginaceae Veronica beccabunga 0.14 0.01 0.06 0.01 0.86 0.08 0.63 0.05 1.70 0.15 5 ± ± ± ± ± Primulaceae Lysimachia thyrsiflora 0.08 0.03 0.69 0.10 0.95 0.09 0.51 0.11 1.68 0.26 4 ± ± ± ± ± Lysimachia vulgaris 0.23 0.02 0.43 0.03 1.00 0.11 - - 1.62 0.19 5 ± ± ± ± Rosaceae Comarum palustre 0.14 0.05 0.41 0.22 0.37 0.11 0.13 0.04 1.06 0.38 4 ± ± ± ± ± a Origin of plant material: 1—Purchased from Vegtech, seeds collected in Sweden; 2—Rådmansö, 59◦440 N 18◦56”’ E; 3—Flemingsberg, 59◦13”’ N 17◦59”’ E; 4—Jumkil, 59◦570 N 17◦17”’ E; 5—Norra Djurgården, 59◦210 N 18◦040 E; 6—cultivated from seeds collected at Kristineberg, 65◦040 N 18◦440 E.
Details
-
File Typepdf
-
Upload Time-
-
Content LanguagesEnglish
-
Upload UserAnonymous/Not logged-in
-
File Pages12 Page
-
File Size-