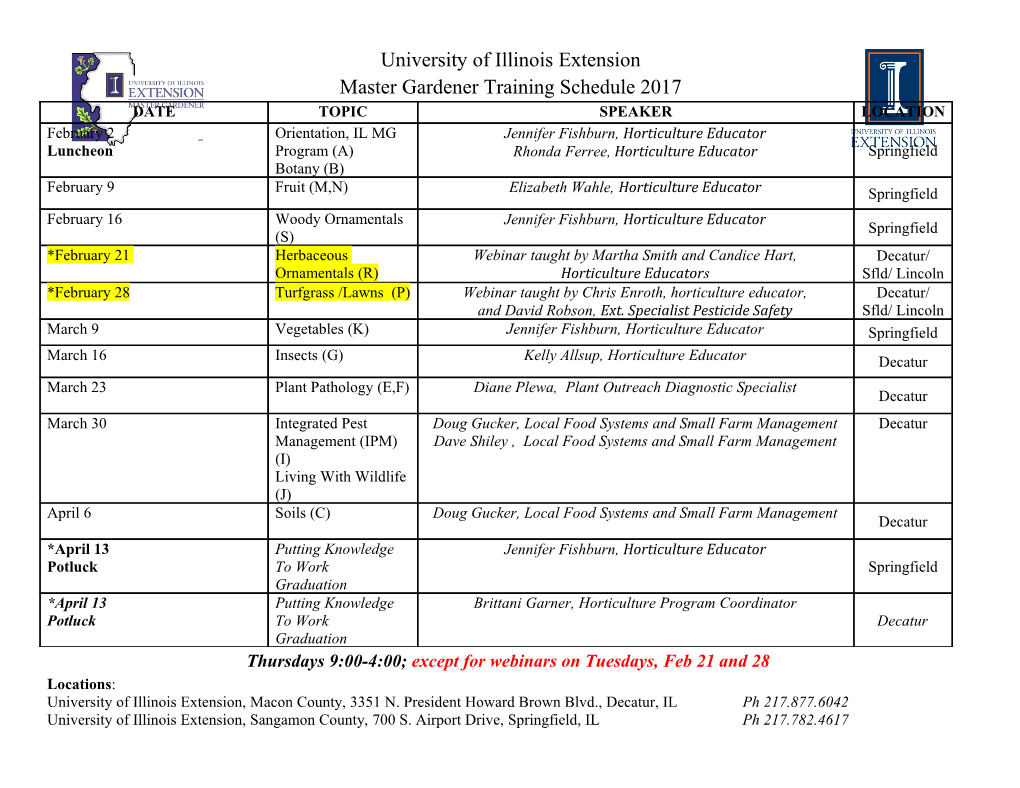
Gravitational probes of dark matter physics Matthew R. Buckleya, Annika H. G. Peterb,c,∗ aDepartment of Physics and Astronomy, Rutgers University, Piscataway, NJ 08854, USA bCCAPP and Department of Physics, The Ohio State University, 191 W. Woodruff Ave., Columbus, OH 43210, USA cDepartment of Astronomy, The Ohio State University, 140 W. 18th Ave., Columbus, OH 43210, USA Abstract The nature of dark matter is one of the most pressing questions in physics. Yet all our present knowledge of the dark sector to date comes from its gravitational interactions with astrophysical systems. Moreover, astronomical results still have immense potential to constrain the particle properties of dark matter in the near future. We introduce a simple 2D parameter space which classifies models in terms of a particle physics interaction strength and a characteristic astrophysical scale on which new physics appears, in order to facilitate communication between the fields of particle physics and astronomy. We survey the known astrophysical anomalies that are suggestive of non-trivial dark matter particle physics, and present a theo- retical and observational program for future astrophysical measurements that will shed light on the nature of dark matter. Keywords: dark matter, galaxies, cosmology, particles PACS: 95.35.+d, 98.62.-g, 98.80.-k, 14.80.-j 1. Introduction For particle physicists, dark matter is clear evidence of the existence of new physics beyond the Standard Model. Significant theoretical effort has been applied to construct viable models of particle dark matter, and the resulting model-space is enormous. While many of these models contain particles which are \minimal" dark matter candidates in the cosmological sense (i.e., cold, functionally collisionless, non-baryonic matter), many others contain dark matter candidates that deviate at some level from the cold dark matter (CDM) paradigm [1, 2, 3]. Well known examples include warm dark matter (WDM) [4, 5] and self-interacting dark matter (SIDM) [6]. Given the variety of models available, experimental input is necessary to narrow down the possibilities. While many experiments have searched for signals of particle dark matter using a variety of traditional particle physics techniques [7, 8, 9] (e.g., collider production [10, 11, 12], direct detection [13, 14, 15, 16, 17], and indirect detection [18, 19, 20]), so far all results have been negative. Consequentially, the primary source of positive statements about the microphysical properties of dark matter continues to be the astrophysical and cosmological study of dark matter's gravitational interactions. This is perhaps not surprising. After all, it was through gravity that dark matter was discovered [1, 21, 22], and its status as a non-relativistic (\cold," or at least no more than \warm") component of the Universe at the time of structure-formation verified [23, 24, 25]. As astronomical surveys and galaxy simulations continue to improve, so too will our ability to explore the distribution of dark matter in the Universe and potentially arXiv:1712.06615v3 [astro-ph.CO] 26 Sep 2018 discover deviations from the CDM paradigm, as predicted by many of the theoretical dark matter models. In many cases these astrophysics techniques are sensitive to dark matter properties orthogonal to those measured by particle physics experiments. Specifically, traditional particle physics techniques probe the microphysical interactions between dark matter and the Standard Model. However, the distribution of dark ∗Principal corresponding author Email addresses: [email protected] (Matthew R. Buckley), [email protected] (Annika H. G. Peter) Preprint submitted to Elsevier September 27, 2018 matter in the Universe may be governed by additional interactions within a \dark sector" that are secluded from the Standard Model, in addition to interactions between dark matter and the Standard Model. Such interactions would not appear in experiments probing the Standard Model-dark matter scattering, but would appear in astrophysical measurements by altering the spatial distribution of dark matter in the Universe with respect to the CDM prediction. The microphysical properties of dark matter | particle mass, number of species, interaction strength of each particle with Standard Model and dark particles | can imprint themselves on the macroscopic distribution of dark matter in the Universe. To illustrate the power of astrophysics to reveal the microphysical properties of dark matter, it is useful to consider the decidedly non-minimal Standard Model. For example, because the Standard Model includes charged particles and photons, gas can cool. This enables the formation of stars and galaxies. One might imagine that hypothetical researchers in the dark sector would be able to identify at least some of the major components and non-gravitational forces of the Standard Model based on gravitational evidence of the clustering of Standard Model particles. We explore this thought experiment in more detail in Section 2 of this work. As this conceptual exercise will demonstrate, astronomical observations constitute measurements of dark matter microphysics. There is immense potential for discovery in the intersection of particle and astrophysical measurements of dark matter. Positive signals of deviations from the gravitational predictions of CDM would give the- oretical physicists much-needed experimental guidance about parameters that are not easily measured in particle physics experiments. If, on the other hand, all astrophysical studies of dark matter were to find correspondence with the CDM predictions, the improved knowledge of dark matter distributions would be extremely useful for reducing major sources of theoretical uncertainties in the particle physics experiments [26, 27, 28, 29, 30]. Likewise, results from particle experiments can either restrict the possible model space relevant to novel astrophysical signals, or suggest specific deviations from the CDM paradigm which can be confirmed by later surveys. Though the connection between astrophysical measurements and particle physics models of dark matter is recognized for particular models (notably, sterile neutrinos [31, 32, 33, 34, 35]), the full potential is perhaps not appreciated in the broader context. This is in part due to the separate languages used by particle physicists and astrophysicists to describe dark matter, and in part due to the fact that astrophysical and particle-physics measurements of dark matter often depend on very different subsets of the parameter space of dark matter microphysics. Finally, the \natural" set of metrics used to constrain or define dark matter depend strongly on the type of experiment or observation used to find dark matter. We have two primary goals for this paper. First, we want to present a compact parameter space to capture the phenomenology of dark matter models relevant to both particle physicists and astrophysicists. To that end, we propose a simple two-dimensional parameter space, with one axis closely tied to particle dark matter searches and the other closely tied to astronomical searches, to orient dark-matter hunters in both fields. We show where models of current interest lie in this space, and where potential discrepancies between observation and CDM predictions are located. Second, we advocate a long-term plan for sharpening astrophysical constraints on dark-matter microphysics. This project will require close collaboration between physicists and astronomers (many of whom may not have dark matter as a primary area of research), which motivates our simple parameter space which translates between the two fields. The two-dimensional description is a simple mapping which can be applied to a wide range of models and makes clear the sensitivity of both particle- and astrophysical-probes to the dark sector. By considering where specific models fall in this parameter space, particle physicists may identify new constraints or observables from astrophysics, while astrophysicists may find applications for their results of which they were previously unaware. The two parameters we adopt are: 1. The characteristic interaction strength between dark matter and the Standard Model, Λ−1; and 2. The characteristic largest dark matter halo mass where significant deviations from CDM predictions should arise, Mhalo. The halo is the fundamental unit of cosmological dark matter on non-linear scales, a self-gravitating collection of dark-matter particles in equilibrium formed when a perturbation in the homogeneous universe detaches from the Hubble flow, and the structure within which galaxies live. 2 The former parameter (defined to have dimensions of inverse energy) gives an estimate of the ability of particle physics experiments to discover dark matter through interactions with baryonic (used in the astro- nomical sense: all Standard Model particles but neutrinos and photons) matter. The latter gives an estimate of the type of astrophysical system whose dynamics must be understood in order to discover the presence of unique dark matter physics. We emphasize that these two parameters are not intended to encompass the full behavior from a particular model of dark matter. Given the breadth of theoretical work on this topic, many well-motivated models of dark matter can have unique phenomenology that would be difficult to capture in any low-dimensional set of parameters. However, flattening the model space down to these two parameters allows for direct comparison of a variety of models, which is particularly
Details
-
File Typepdf
-
Upload Time-
-
Content LanguagesEnglish
-
Upload UserAnonymous/Not logged-in
-
File Pages77 Page
-
File Size-