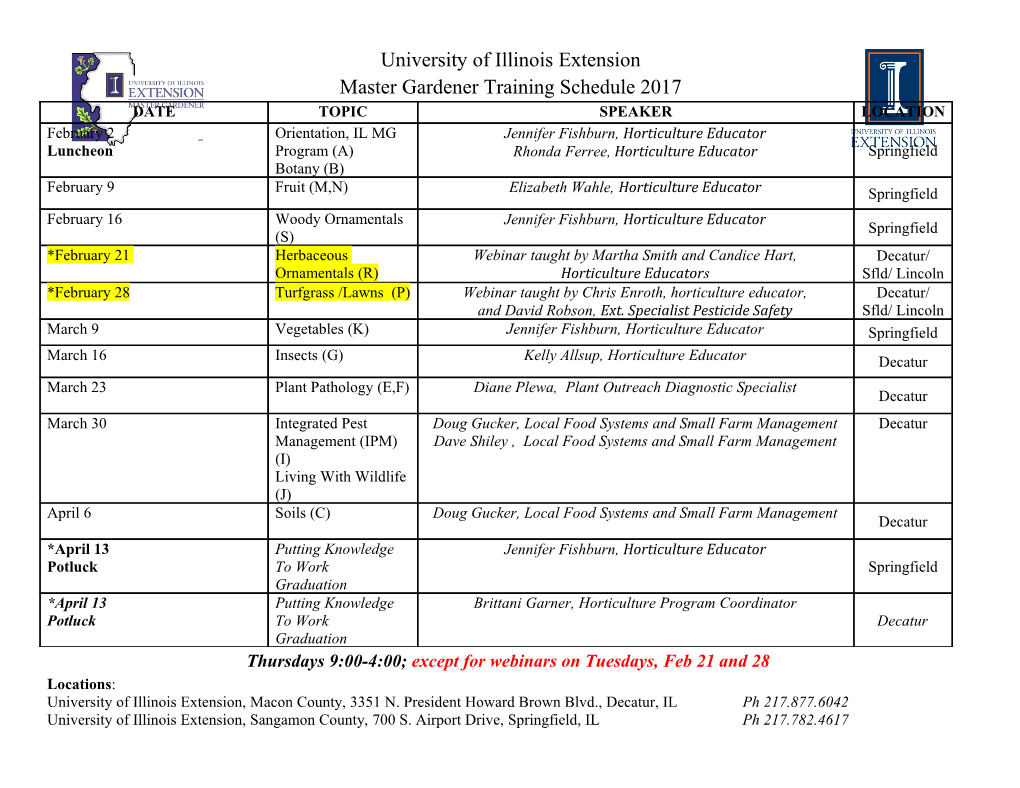
Asymmetric catalysis in complex target synthesis Mark S. Taylor and Eric N. Jacobsen* Department of Chemistry and Chemical Biology, Harvard University, Cambridge, MA 02138 Edited by Jack Halpern, University of Chicago, Chicago, IL, and approved January 30, 2004 (received for review November 26, 2003) This article describes three distinct strategies by which stereochemically complex molecules are synthesized and the ways asymmetric ca- talysis can impact on all three. The development of general methods to prepare synthetically useful building blocks leads to an expanded ‘‘chiral pool’’ of potential starting materials for asymmetric synthesis. The possibility of discovering new reactions to access new types of building blocks is particularly attractive and serves to help define the frontiers of the field. Asymmetric catalysis can also be applied to di- astereoselective synthesis such that the stereochemistry of the catalyst, and not that of the substrate, determines the relative configura- tion of the product. Finally, in reactions where multiple stereocenters are generated simultaneously or in tandem, catalyst and substrate control can operate in a complementary manner to achieve one of many possible stereochemical outcomes selectively. he synthesis of both natural and unnatural organic compounds in optically active form is a cen- T tral challenge in chemistry, es- pecially in relation to the study of bio- logically active compounds. Natural products and synthetic pharmaceutical agents display a rich diversity of molecu- lar structures that range from very sim- ple to astonishingly intricate. A feature common to many such compounds is the presence of multiple stereocenters, giv- Scheme 1. Masamune–Sharpless synthesis of the L-hexoses. Asterisks denote stereocenters under ing rise to the possibility of large num- absolute control. bers of stereoisomers (2n, where n is the number of stereocenters). The control of the relationship between stereo- substrate control. Other methods for rap- With the discovery of the first general centers is therefore an essential element idly achieving stereochemical complexity asymmetric synthetic reactions in the to the synthesis of many complex targets under catalyst control are possible, includ- 1980s, the way that stereochemically com- of interest. ing the desymmetrization of meso com- plex targets could be accessed was ex- There are several ways to generate ste- pounds and stereospecific reactions of panded in two crucial ways. Asymmetric reochemical complexity in a selective geometrically defined precursors. synthesis began to provide access to a manner (Fig. 1). Chiral building block Before the advent of efficient asymmet- greatly expanded pool of readily available assembly, for which peptide synthesis rep- ric synthetic methods, application of the chiral starting materials (2), and synthetic resents the prototype, relies on the avail- chiral building block approach was con- chemists could now devise strategies to ability of the appropriate optically pure strained by the very limited pool of opti- prepare target structures based on chiral starting materials along with the reactions cally active compounds provided by na- starting materials unavailable from nature. to elaborate and couple them. An alterna- ture in abundance (the ‘‘chiral pool’’) (1). In addition, ‘‘powerful’’ chiral reagents tive is diastereoselective synthesis, wherein Because of this constraint, strategies in and catalysts were identified that can in- new stereocenters are introduced into target-oriented synthesis were largely pre- duce highly diastereoselective reactions compounds bearing preexisting ones. The through external stereocontrol, overcom- selectivity may arise either from the prop- mised on the fact that optically active ing or complementing the inherent sub- erties inherent to the substrate (substrate starting materials were particularly pre- strate bias (3). control) or from an external agent (re- cious, and the application of substrate- Masamune and Sharpless provided a agent or catalyst control). A third strategy controlled diastereoselective processes was involves the use of transformations that emphasized. Although this now-classic seminal and brilliant illustration of the simultaneously introduce multiple stereo- approach has proven enormously power- potential of catalyst-induced double ste- centers in a single operation. For example, ful and will surely always play a critical reodifferentiation in natural product syn- the coupling of two prochiral substrates role in complex molecule construction, it thesis in their stereocontrolled synthesis of may result in four (or more) stereochemi- is also inherently limited because it re- the L-hexoses. The then-recently devel- cal outcomes; enantio- and diastereoselec- quires a specific design concept for every oped titanium tartrate (Sharpless) epoxi- tive variants of such processes usually in- new target (including diastereomers of the dation catalyst was used to generate the corporate elements of catalyst and same target). key chiral starting material and was subse- quently applied in the diastereoselective elaboration of a late-stage intermediate This paper was submitted directly (Track II) to the PNAS office. Abbreviation: HDA, hetero-Diels–Alder. *To whom correspondence should be addressed. E-mail: Fig. 1. Strategies for the construction of stereochemically complex targets. Asterisks denote the [email protected]. presence of defined stereocenters. © 2004 by The National Academy of Sciences of the USA 5368–5373 ͉ PNAS ͉ April 13, 2004 ͉ vol. 101 ͉ no. 15 www.pnas.org͞cgi͞doi͞10.1073͞pnas.0307893101 Downloaded by guest on September 30, 2021 SPECIAL FEATURE PERSPECTIVE Fig. 2. Selected applications of the HKR reaction in natural product synthesis. (Scheme 1) (4). Ultimately, all four con- metric catalytic reactions. The fruit of this many cases, this has forced synthetic tiguous stereocenters were controlled in- effort has been the recent discovery of a chemists to develop lengthy, inefficient dependently, providing access to each of variety of remarkably general catalytic syntheses to make use of available the eight hexose diastereomers by essen- oxidation, reduction, and C–C bond-form- compounds. tially the same route. ing reactions (5). The impact of these Asymmetric catalysis can provide a The strategy underlying the synthesis of methods on the manner in which complex powerful solution to this problem by ren- the hexoses is so compelling that one targets can be synthesized is just begin- dering accessible chiral materials not might ask why asymmetric catalysis has ning to be felt (6) and is discussed here in readily provided by nature. In principle, not become the approach by which all several representative examples. small molecule synthetic catalysts can be complex target synthesis is undertaken. applied to the synthesis of building blocks One answer is that certain targets are best An Expanded Chiral Pool as either enantiomer and with nearly com- accessed from nature’s chiral pool or are The chiral pool approach to synthesis of plete generality. For example, whereas well suited to substrate-controlled diaste- optically active targets can be extremely nature’s chiral pool is not a useful source reoselective synthesis strategies, and asym- attractive if nature happens to provide of optically active terminal epoxides, these metric catalytic methods do not offer any an abundant supply of a starting mate- very useful compounds are now readily advantage. However, another reason is rial appropriate for the synthetic target. accessible in one step from the corre- that the hexose synthesis relied on a reac- Accordingly, considerable effort and sponding racemates by the (salen)Co- tion that was far ahead of its time, and creativity has been directed toward us- catalyzed hydrolytic kinetic resolution (9). that there are still too few reactions as ing inexpensive chiral pool elements in As a result, it is now possible to evaluate effective and as general as the Sharpless target-oriented synthesis. In the best complex targets strategically with the epoxidation. As a result, it has not been cases, some of the most practical and knowledge that enantiopure terminal ep- possible to devise attractive synthetic brilliant syntheses ever devised have oxides represent viable starting points. routes to target structures based on avail- used this approach. The Stork syntheses Various groups have incorporated this ␣ able methods. of prostaglandin A2 and F2 are classic recently developed transformation into The latter consideration has motivated examples (7, 8). However, nature pro- synthetic routes to natural products of several research groups throughout the vides only a limited number of chiral varying complexity (Fig. 2) (10–13). world to overcome this limitation, by ex- starting materials, and usually only one In many cases, retrosynthetic analysis of panding the availability of powerful asym- enantiomer is produced naturally. In a complex target leads to chiral starting Scheme 2. Synthesis of the central fragment of FR901464. Taylor and Jacobsen PNAS ͉ April 13, 2004 ͉ vol. 101 ͉ no. 15 ͉ 5369 Downloaded by guest on September 30, 2021 Scheme 3. Catalyst-controlled synthesis of iridoid natural products. Scheme 4. Catalyst-controlled syntheses of manzacidin A and C. materials that are not readily accessible by thetic chemists no longer must work only FR901464 synthesis suggest the feasibility available methods. In these situations, nat- with what nature provides
Details
-
File Typepdf
-
Upload Time-
-
Content LanguagesEnglish
-
Upload UserAnonymous/Not logged-in
-
File Pages6 Page
-
File Size-