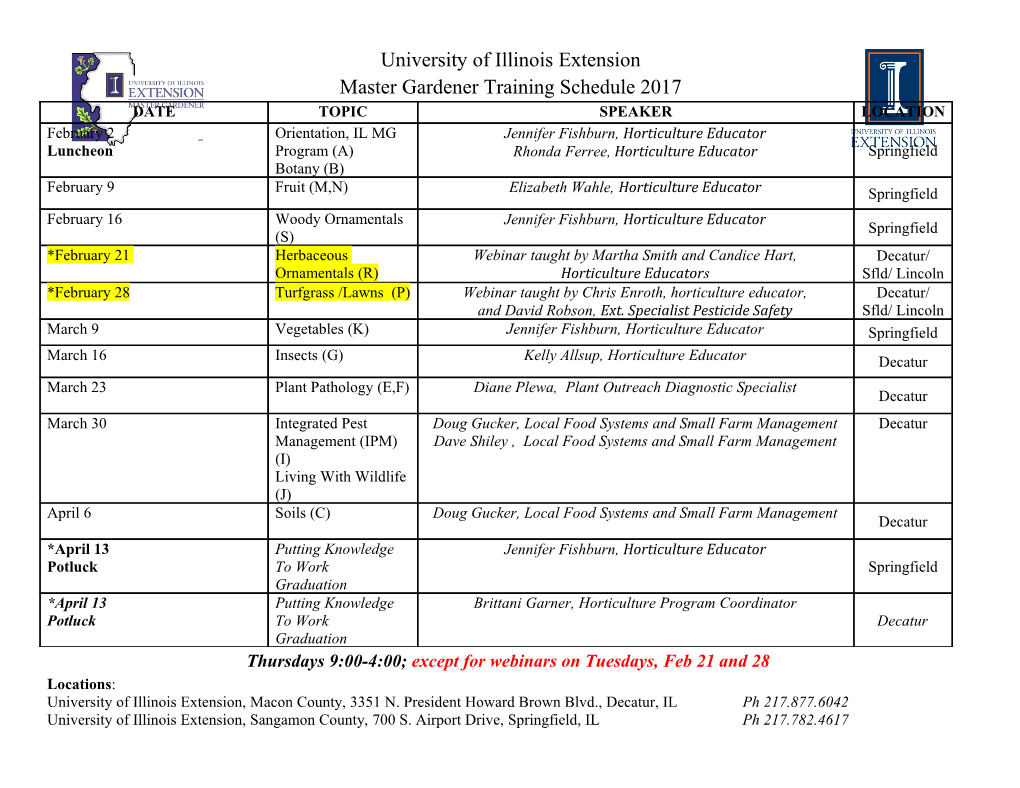
ASHWIN JS UNIT V PHOTO ELECTRIC DEVICE PHOTO EMISSIVITY Using the experimental arrangement indicated in Fig. 19-1, the following characteristics of the photoemissive effect are obtained: The photoelectrons liberated from the photosensitive surface possess a range of initial velocities. However, & definite negative potential when applied between the collector and the emitting surface will retard the fastest-moving electrons. This indicates that the emitted electrons are liberated with all velocities from zero to a definite maximum value. The maximum velocity of the emitted electrons is 2 1/2mv max =evr where Vr, is the retarding potential, in volts, necessary to reduce the photo current to zero. As the accelerating potential is increased, the number of electrons to the collector increases until saturation occurs. In Fig. 19-2a are plotted curves showing the variation of photocurrent I versus anode potential V with the light intensity j as a parameter. These curves indicate that V,, and hence Vmax, are independent of the light intensity. 2. If the photoelectric current is measured as a function of the anode potential for different light frequencies f and equal intensities of the incident light, the results obtained are essentially those illustrated in Fig. 19-26 It is observed that the greater the frequency of the incident light, the greater must be the retarding potential to reduce the photocurrent to zero. This means, of course, that the maximum velocity of emission of the photoelectrons increases with the frequency of the incident light. Experimentally, it is found that a linear relationship exists between V, and f. The experimental facts 1 and 2 may be summarized in the statement that the maximum energy of the electrons liberated photoelectrically is independent of the light intensity but varies linearly with the frequency of the incident light. 3. If the saturation current is plotted as a function of the light intensity, we find that the photoelectric current is directly proportional to the intensity of the light ASHWIN JS 4. The foregoing photoelectric characteristics are practically independent of temperature, within wide ranges of temperature. 5. The electrons are emitted immediately upon the exposure of the surface to light. The time lag has been determined experimentally to be less than 3nsec. 6 Photoelectric cells are selective devices. This means that a given intensity of light of one wavelength, say red light, will not liberate the same number of electrons as an equal intensity of light of another wavelength. Say blue light. That is, the photoelectric yield, defined as the photocurrent (in amperes) per watt of incident light, depends upon the frequency of the light. PHOTOELECTRIC THEORY The foregoing experimental facts find their explanation in the electronic theory of metals and in the light-quantum hypothesis of Planck. As discussed in Planck made the fundamental assumption that radiant energy is not continuous, but can exist only in discrete quantities called quanta, or photons. Bohr used the same theory of photons to explain the spectra of atoms . Einstein applied the same hypothesis to explain photo emission, as we now demonstrate. Planck's basic assumption is that, associated with light of frequency f (hertz) are a number of photons, each of which has an energy hf (joules), The greater the intensity of the light, the larger the number of photons present, but the energy of each photon remains unchanged. Of course, if the light beam is heterogeneous rather than monochromatic, the energy of the photons therewith associated will vary and will depend upon the frequency. • According to Einstein, each photon of a light wave of frequency has the energy E is given by, • E=hf • where E= energy of photon(joule) • h= planks constant-6.626 x 10-34J-s • f= frequency of photon(Hz) • Einstein Equation 2 1/2mv hf-Uw ASHWIN JS LAW OF PHOTO ELECTRIC EFFECT • Photoelectric effect is directly proportional to intensity. • If the frequency of the incident light is less than the threshold frequency then no electron ejected, no matter what the intensity . • The maximum kinetic energy of the electrons depend on the frequency of the incident light. • The electrons were emitted immediately - no time lag. PHOTOTUBE A phototube or photoelectric cell is a type of gas-filled or vacuum tube that is sensitive to light. Such a tube is more correctly called a 'photoemissive cell' OPERATING PRINCIPLES Phototubes operate according to the photoelectric effect: Incoming photons strike a photocathode, knocking electrons out of its surface, which are attracted to an anode. Thus current is dependent on the frequency and intensity of incoming photons. Unlike photomultiplier tubes, no amplification takes place, so the current through the device is typically of the order of a few microamperes. Vacuum devices have a near constant anode current for a given level of illumination relative to anode voltage. Gas filled devices are more sensitive but the frequency response to modulated illumination falls off at lower frequencies compared to the vacuum devices. APPLICATIONS One major application of the phototube was the reading of optical sound tracks for projected films. Phototubes were used in a variety of light-sensing applications until they were superseded by photoresistors and photodiodes ASHWIN JS PHOTO MULTIPLIER TUBE The photo multiplier tube consists of an evacuated glass envelope containing a photo cathode, an anode and several additional electrodes, termed Dynodes, each at a higher voltage, than the previous dynode. Figure 13.32 illustrates the principle of the photo multiplier. Electrons emitted by the cathode are attracted to the first dynode. Here a phenomenon known as secondary emission takes place. When electrons moving at a high velocity strike an appropriate material, the material emits a greater number of electrons than it was struck with. In this device, the high velocity is achieved by the use of a high voltage between the anode and the cathode. The electrons emitted by the first dynode are then attracted to the second dynode, where the same action takes place again. Each dynode is at a higher voltage, in order to achieve the requisite electron velocity each time. Hence, secondary emission, and a resulting electron multiplication, occurs at each step, with an overall increase in electron flow that may be very great. Amplification of the original current by much as 105 — 109 is common. Luminons sensitivities range from lA per lumen or less, to over 2000 A per lumen. Typical anode current ratings range from a minimum of 100 μA to a maximum of 1 mA. The extreme luminous sensitivity possible with these devices is such that for a sensitivity of 100 A per lumen, only 10-5 lumen is needed to produce 1 mA of output current. Magnetic fields affect the photo multiplier because some electrons may be deflected from their normal path between stages and therefore never reach a dynode or anode. Hence, the gain falls. To minimise this effect μ-metal magnetic shields are often placed around the photo multiplier tube. PHOTOCONDUCTIVITY Photoconductivity is an optical and electrical phenomenon in which a material becomes more electrically conductive due to the absorption of electromagnetic radiation such as visible light, ultraviolet light, infrared light, or gamma radiation When light is absorbed by a material such as a semiconductor, the number of free electrons and electron holes increases and raises its electrical conductivity. To cause excitation, the light that strikes the semiconductor must have enough energy to raise electrons across the band gap, or to excite the impurities within the band gap. When a bias voltage and a load resistor are used in series with the semiconductor, a voltage drop across the load resistors can be measured when the change in electrical conductivity of the material varies the current through the circuit. Classic examples of photoconductive materials include : photocopying (xerography); lead sulfide, used in infrared detection applications Application When a photoconductive material is connected as part of a circuit, it functions as a resistor whose resistance depends on the light intensity. In this context, the material is called a photoresistor (also called light-dependent resistor or photoconductor). The most common ASHWIN JS application of photoresistors is as photodetectors, i.e. devices that measure light intensity. Photoresistors are not the only type of photodetector—other types include charge-coupled devices (CCDs), photodiodes and phototransistors—but they are among the most common. Some photodetector applications in which photoresistors are often used include camera light meters, street lights, clock radios, infrared detectors, nanophotonic systems and low-dimensional photo- sensors devices PHOTOVOLTAIC EFFECT The photovoltaic effect is the creation of voltage and electric current in a material upon exposure to light and is a physical and chemical phenomenon. The photovoltaic effect is closely related to the photoelectric effect. In either case, light is absorbed, causing excitation of an electron or other charge carrier to a higher-energy state. The main distinction is that the term photoelectric effect is now usually used when the electron is ejected out of the material (usually into a vacuum) and photovoltaic effect used when the excited charge carrier is still contained within the material. In either case, an electric potential (or voltage) is produced by the separation of charges, and the light has to
Details
-
File Typepdf
-
Upload Time-
-
Content LanguagesEnglish
-
Upload UserAnonymous/Not logged-in
-
File Pages16 Page
-
File Size-