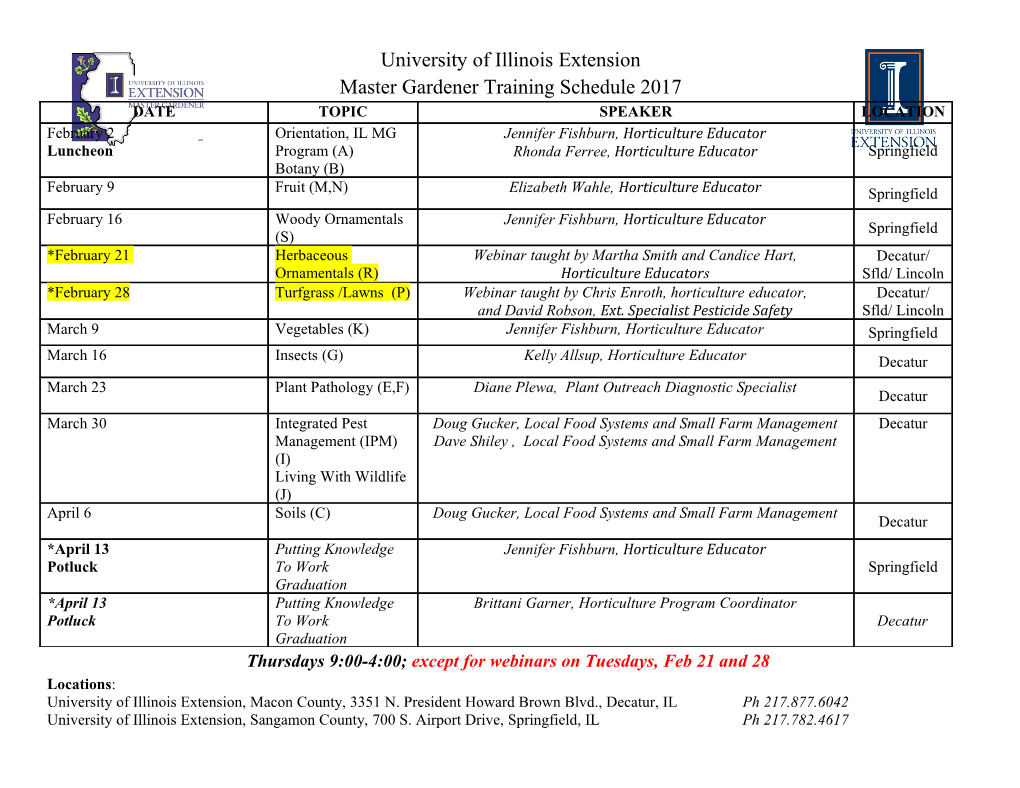
Graduate Theses, Dissertations, and Problem Reports 2018 Proteasomal ATPases Hard at Work: The Inner Workings of a Protein Destruction Machine. Aaron M. Snoberger Follow this and additional works at: https://researchrepository.wvu.edu/etd Recommended Citation Snoberger, Aaron M., "Proteasomal ATPases Hard at Work: The Inner Workings of a Protein Destruction Machine." (2018). Graduate Theses, Dissertations, and Problem Reports. 8186. https://researchrepository.wvu.edu/etd/8186 This Dissertation is protected by copyright and/or related rights. It has been brought to you by the The Research Repository @ WVU with permission from the rights-holder(s). You are free to use this Dissertation in any way that is permitted by the copyright and related rights legislation that applies to your use. For other uses you must obtain permission from the rights-holder(s) directly, unless additional rights are indicated by a Creative Commons license in the record and/ or on the work itself. This Dissertation has been accepted for inclusion in WVU Graduate Theses, Dissertations, and Problem Reports collection by an authorized administrator of The Research Repository @ WVU. For more information, please contact [email protected]. Title Page Proteasomal ATPases Hard at Work: The Inner Workings of a Protein Destruction Machine Aaron M. Snoberger Dissertation submitted to the School of Medicine at West Virginia University in partial fulfillment of the requirements for the degree of Doctor of Philosophy in Biochemistry and Molecular Biology David M. Smith, Ph.D., Chair Michael D. Schaller, Ph.D. Maxim Sokolov, Ph.D. Michael R. Gunther, Ph.D. David P. Siderovski, Ph.D. Department of Biochemistry Morgantown, West Virginia 2018 Keywords: proteasome, molecular motor, Rpt, ATPase, AAA, AAA+ Copyright 2018 Aaron M. Snoberger Abstract Proteasomal ATPases Hard at Work: The Inner Workings of a Protein Destruction Machine Aaron M. Snoberger Across all domains of life, the proteasome is responsible for the majority of targeted protein degradation in the cell. Often, the proteasome is thought of as the molecular “garbageman” of the cell. While it is true that the proteasome degrades and eliminates misfolded proteins, the proteasome is also capable of degrading fully folded, functional proteins whose presence is no longer required (e.g. during embryonic development, cell cycle changes, etc.). Despite its crucial role in virtually every cellular process, our understanding of how the proteasome operates from a mechanistic perspective is still highly limited. In order to prevent unregulated degradation the protease sites of the proteasome are sequestered inside its hollow interior. While loosely folded proteins can enter the degradation chamber without the requirement of energy, proteins with secondary structure can only be degraded when they are properly recognized (e.g. by ubiquitin tags), unfolded, and injected into the protease chamber for degradation. Protein recognition, unfolding, and injection into the protease chamber all depend on ATP. However, very little is known about how such chemical energy is converted to mechanical work. In this dissertation we sought to understand the logistics of nucleotide binding and hydrolysis, and also to determine the conformational changes that can regulate protein entry and degradation by the proteasome. To this end, we focused on one of the most common regulators from eukaryotes-- the heterohexameric 19S ATPases, as well as its homohexameric archaeal homolog-- “PAN” (proteasome activating nucleotidase). Based on our extensive analysis, our data support a neighbor-binding sequential hydrolysis mechanism for the proteasomal ATPases. Furthermore, we show that these ATPases are highly processive, even when they reach more tightly folded domains of a protein, which is unlike what had been proposed previously based on studies of other ATP-dependent proteases (e.g. ClpX, which often “slips” and “stalls” at these more tightly folded domains). This tight binding of the proteasomal ATPases appears to be due to its crucial trans-arginine fingers that “sense” bound nucleotides in its neighboring subunit (which ClpX lacks), and we propose that this processivity arose due to the diverse client proteins the proteasome must encounter (e.g. it must engage and unfold many types of proteins, even ones that are fully folded and functional). Lastly, we have developed a disulfide engineering approach to show that PAN’s N-terminal domains adopt distinct conformations that set the rate of ATP hydrolysis. This novel approach has allowed us to isolate specific subunits from a homohexamer that are identical in their amino acid sequence, but that adopt different conformations when they form a hexameric ring. This disulfide engineering approach we’ve developed is a powerful method to analyze structural asymmetries in homomeric protein complexes with minimal structural perturbations, which has not been accomplished before and opens the door to an entire new approach to studying the function of the molecular motors. We started this work with the goal of understanding the logistics of the mechano-chemical cycle of the proteasomal ATPases. Indeed, we have developed novel methods to better understand the inner workings of this complex multimeric machine, and the groundwork we lay here has contributed greatly to our knowledge of the proteasomal ATPases, and will also push forward our understanding of other AAA+ ATPases and molecular motors in general. Ultimately, a better understanding of these complex machines will aid in the development of new therapies to combat diseases where these machines are dysregulated. Dedication “It’s easier to steer a moving ship” To my family and friends who encouraged me to keep moving, even in those times that I didn’t know what I was moving toward. It’s always easier to change course if you don’t stagnate, a philosophy that’s proven just as relevant in the ‘science world’ as it has in the ‘real world’. To Jess, my best friend, there’s no other co-pilot I’d rather share this crazy journey with. iii Acknowledgments Thanks to my graduate mentor, David Smith, for guiding me through this process. You’ve given me the freedom to pursue projects I’m passionate about, and your mentoring style has helped me to grow and be independent in ways that many don’t experience until their post-docs. I couldn’t have asked for a better mentor-- you’ve been patient enough to let me learn from my mistakes, yet have always been there to lend help when I need it. My wife and I are also grateful for how you lead by example-- you always work hard, yet always find time for what is most important in life. You’ve truly been a great mentor in every sense of the word. Thanks to Rob Wysolmerski, my undergraduate research advisor, for introducing me to the fascinating world of biomedical research. Thanks to my committee members, Michael Schaller, Max Sokolov, Michael Gunther, Dave Siderovski, and former member Bill Wonderlin for guiding my research. Thanks to the members of the “old guys” journal club for challenging me and helping to develop my critical reading of the literature. Thanks to Evan Brettrager for substantially increasing my productivity in your time here—with your help I had the time to pursue the more risky projects that turned out to be quite fruitful, and thanks to Taylor Thomas for carrying on this exciting research after my departure. Lastly, thanks to present and past members of the Smith Lab for making this journey an enjoyable one: Jane Schupp, Tiffany Thibaudeau, Young-Chan Kim, Evan Brettrager, Ray Anderson, and Taylor Thomas. iv Table of Contents Title Page ..................................................................................................................................... i Abstract....................................................................................................................................... ii Dedication .................................................................................................................................. iii Acknowledgments ...................................................................................................................... iv List of Tables ........................................................................................................................... viii List of Figures ............................................................................................................................ ix List of Abbreviations ................................................................................................................... xi Chapter 1: Review of Literature ................................................................................................. 1 Introduction ............................................................................................................................ 2 Proteasomal ATPases from other species are closely related to the 19S ............................... 4 The role of the CC and OB domains ....................................................................................... 5 The role of the AAA+ ATPase domain in proteasomal ATPases ............................................. 5 How do substrates stimulate their own degradation: A role for the CC domains? ................... 8 CC domains............................................................................................................................ 9 The role of CCs in substrate binding ......................................................................................11
Details
-
File Typepdf
-
Upload Time-
-
Content LanguagesEnglish
-
Upload UserAnonymous/Not logged-in
-
File Pages200 Page
-
File Size-