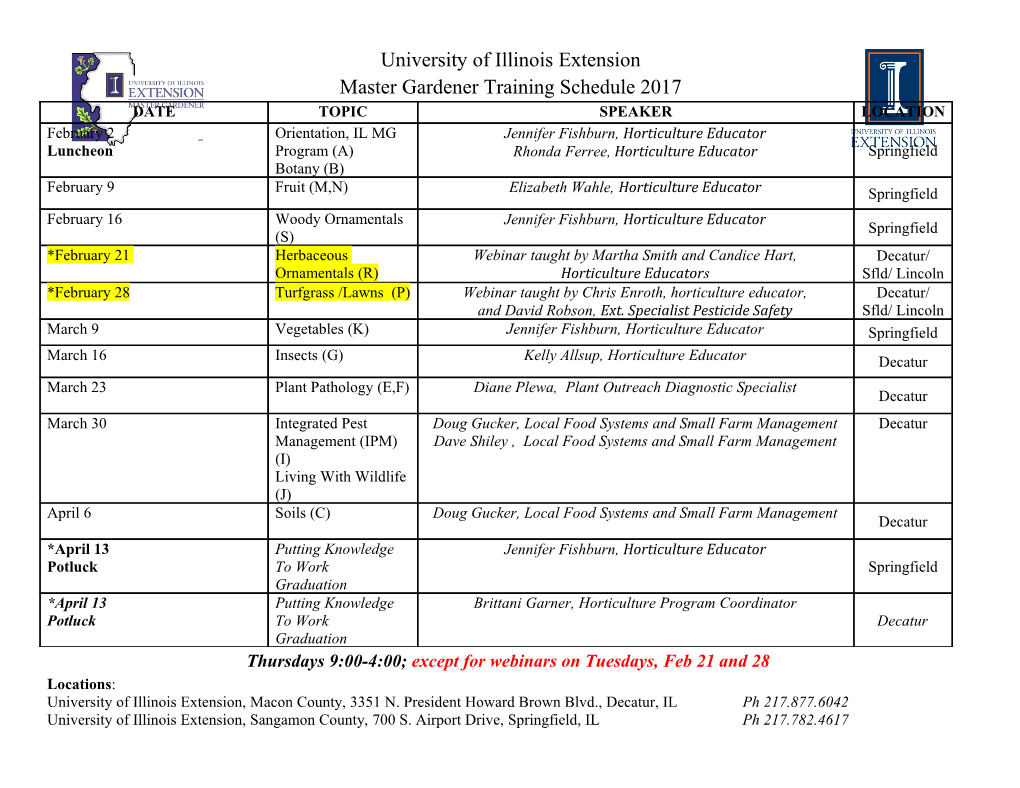
R EPORTS as evidence for at least some surficial H2O(11). Both polar regions contain layered terrains that Seasonal Variations of Snow are characterized by about 3 km of relief (5, 12) and are believed to be composed primarily of Depth on Mars H2O ice mixed with dust. The residual north polar ice cap correlates well with elevated to- 1 1,2 1,2 David E. Smith, * Maria T. Zuber, Gregory A. Neumann pography that defines the layered terrains (Fig. 1, left) (12). However, elevated layered deposits Using topography collected over one martian year from the Mars Orbiter Laser in the south are much broader in spatial extent Altimeter on the Mars Global Surveyor (MGS) spacecraft, we have measured than the residual ice cap (Fig. 1, right) (5). temporal changes in the elevation of the martian surface that correlate with Using data from the Mars Orbiter Laser the seasonal cycle of carbon dioxide exchange between the surface and at- Altimeter (MOLA) (13) on the Mars Global mosphere. The greatest elevation change (1.5 to 2 meters) occurs at high Surveyor (MGS) (14) spacecraft, we have latitudes ( above 80¡), whereas the bulk of the mass exchange occurs at lower made the first measurements of temporal latitudes (below 75¡ N and below 73¡ S). An unexpected period of sublimation changes in the elevation of the surface of was observed during northern hemisphere autumn, coincident with dust storms Mars and of the planet’s global mass redis- in the southern hemisphere. Analysis of MGS Doppler tracking residuals re- tribution due to the yearly cycle of CO2 vealed temporal variations in the flattening of Mars that correlate with ele- exchange. We analyzed over 400 million el- vation changes. The combined changes in gravity and elevation constrain the evation measurements (15) and over 66 mil- average density of seasonally deposited carbon dioxide to be 910 Ϯ 230 lion altimetric crossovers (16) from MOLA, kilograms per cubic meter, which is considerably denser than terrestrial snow. as well as all MGS X-band Doppler and range tracking (17) observations collected Over the course of its year, Mars exchanges caps and surroundings. Continued deposi- during the MGS mapping and extended mis- up to a third of its carbon dioxide (CO2) tion causes the “frost line” to migrate from sion phases between 28 February 1999 and atmosphere with the surface, resulting in a the polar regions toward the equator, and 25 May 2001 (18). The observations spanned seasonal redistribution of about 50 bil- surface frost reaches mid-latitudes in late more than a full martian year (19) and en- lionths of the mass of the planet. The sea- winter (3). In early spring, as the surface abled us to observe the seasonal pattern re- sonal polar caps consist mainly of CO2 receives more sunlight, surficial CO2 sub- peating the early part of the cycle. In studying frost deposits whose spatial and temporal limates back into the atmosphere, causing seasonal phenomena on Mars, it is customary distributions are controlled primarily by so- the frost line to retreat to high latitudes. to express time in terms of the solar longi- lar insolation (1). In the northern and south- The elevation of the north pole of Mars is 6 tude, Ls, which runs from 0° to 360° over one ϭ ern hemispheres, CO2 begins to condense km lower than the south pole (4, 5). In the north Mars year, and Ls 0° is defined as the out of the atmosphere at high latitudes dur- during the summer, the temperature rises above vernal equinox in the northern hemisphere. ing autumn as the surface encounters in- the CO2 condensation temperature, and dry ice MGS began its global mapping mission at ϭ creasing darkness (Web fig. 1) (2), depos- frost deposited during the previous winter sub- Ls 103°, during northern hemisphere sum- iting a thin veneer of dry ice on the polar limes away, revealing a residual H2O ice cap (6, mer. Our analysis continued into the MGS ϭ 7). In contrast, the southern polar region is extended mapping mission, through Ls colder and has a lower atmospheric pressure, 547°. (We added 360° to L to denote obser- 1Laboratory for Terrestrial Physics, NASA/Goddard s 2 and as a consequence its surface veneer of CO2 vations collected during the second Mars year Space Flight Center, Greenbelt, MD 20771, USA. Mas- ϭ sachusetts Institute of Technology, Cambridge, MA does not completely sublime. A residual dry ice of mapping; for example, Ls 547° is equiv- ϭ 02139Ð4307, USA. surface is observed even in summer (8), al- alent to Ls 187°, which is northern fall.) *To whom correspondence should be addressed. E- though observations of atmospheric water va- We analyzed MOLA profiles of elevation mail: [email protected] por during some years (9, 10) have been cited in over 1200 0.05°-wide (3 km-wide) latitu- Fig. 1. Shaded relief 180˚ 0˚ maps of (left) north 1 50˚ 0˚ 30˚ and (right) south po- 210˚ 33 lar topography of Mars. The projection is polar stereographic 1 6 2 0˚ 0˚ 0˚ from latitude 72¡ to 4 00˚ the poles. The red con- 2 3 tours in each hemi- sphere represent the 2 approximate extent of 2 9 9 7 7 0˚ 0˚ 0˚ the residual ice caps 0˚ (denoted by high albe- do); the blue contours trace regions of ele- vated polar layered terrains. 3 2 00˚ 4 0˚ 0˚ 0˚ 2 6 1 2 33 10˚ 0˚ 0˚ 30˚ 15 0˚ 180˚ www.sciencemag.org SCIENCE VOL 294 7 DECEMBER 2001 2141 R EPORTS dinal annuli between 60°–87°N, 60°–87°S insolation, but it does correlate with the oc- southern hemisphere, the season was winter and, to isolate systematic errors (20), in the currence of several regional dust storms (24). and CO2 was accumulating at mid- to high equatorial band of 5°S to 5°N (21). Within Although these dust storms were located southern latitudes (Fig. 2, C and D). The each annulus, we created a longitude-aver- mostly in the southern hemisphere, they maximum accumulation in the south was ϳ aged reference basal surface by fitting a 25- raised the temperature of the atmosphere at achieved at Ls 155°, relatively earlier by point running median to all points in all Ls mid- to high latitudes in the northern hemi- 10° Ls than in the north. Sublimation in the bins in the annulus (Web fig. 2) (2). We then sphere by Ͼ20° (24). But a temperature in- southern hemisphere during spring may have calculated residuals of individual elevations crease of this magnitude alone cannot explain been aided by atmospheric warming associ- with respect to the basal topographic surface as much sublimation as was observed. Lateral ated with dust storm activity discussed above. (Web fig. 3) (2, 22) and ordered the obser- atmospheric transport or redistribution of sur- Substantial accumulation began again at ϳ vations by the date on which they were col- face frost by wind (or both) are required to about Ls 390°, in early southern fall. lected. We averaged the residuals to the me- explain the magnitude of the off-season ele- In both hemispheres, the minimum zonal dian surface in 15-day bins and removed a vation decrease. Accumulation in the north surface shows a variation of about 10 cm, global systematic contribution to the eleva- resumed as the atmosphere cleared and which we interpret as a measure of our pre- tion change by differencing the signal in each cooled, and reached a longitudinally aver- cision (Fig. 3). Both hemispheres show a ϳ ϳ hemisphere to that at latitude 60° (20). aged maximum of 1.5 m at 86.5°N at Ls quasi-linear latitudinal trend of maximum ac- In a separate analysis of altimetric cross- 345°, during late winter (Table 1). cumulation of about 4 cm per degree of lati- overs (16), we also divided the data into At the beginning of MGS mapping in the tude. In the south, the trend continued to the 15-day temporal bins, using only low-latitude (that is, non–temporally varying) crossovers 1.6 and, to constrain short-period orbital errors, northern northern northern northern northern summer fall winter spring summer crossovers at higher latitudes within a given 1.2 bin. As for the profile analysis, the crossover lat= 86.5 dust 0.8 85.5 storms A method used altimetry; however, this analysis 82.5 was performed independently and provides a 80.5 cross-check of the analysis of the profile data. 0.4 77.5 In order to minimize noise and maximize 0.0 the signal, we averaged over longitude in both approaches to isolate zonal (latitudinal) 90 180 270 360 450 540 variations (20). A formal error analysis yields 1.6 northern northern northern northern northern a vertical accuracy in each 2-week bin of Ϯ 5 summer fall winter spring summer to 6 cm (Table 1), whereas the observed 1.2 lat= 75.5 dust bin-to-bin noise in the observations is Ϯ10 B ) 72.5 storms cm (23). s 0.8 70.5 67.5 In both hemispheres, the greatest high- eter 0.4 65.5 m latitude surface elevations were observed ( h when the polar regions were in darkness, and ∆ 0.0 the lowest elevations were observed when e g 90 180 270 360 450 540 n those areas were sunlit (Fig. 2). In both hemi- a h 1.6 spheres, the maximum elevation occurred in C southern southern southern southern southern late winter, and greater elevation changes winter spring summer fall winter on i t 1.2 were observed with increasing latitude.
Details
-
File Typepdf
-
Upload Time-
-
Content LanguagesEnglish
-
Upload UserAnonymous/Not logged-in
-
File Pages6 Page
-
File Size-