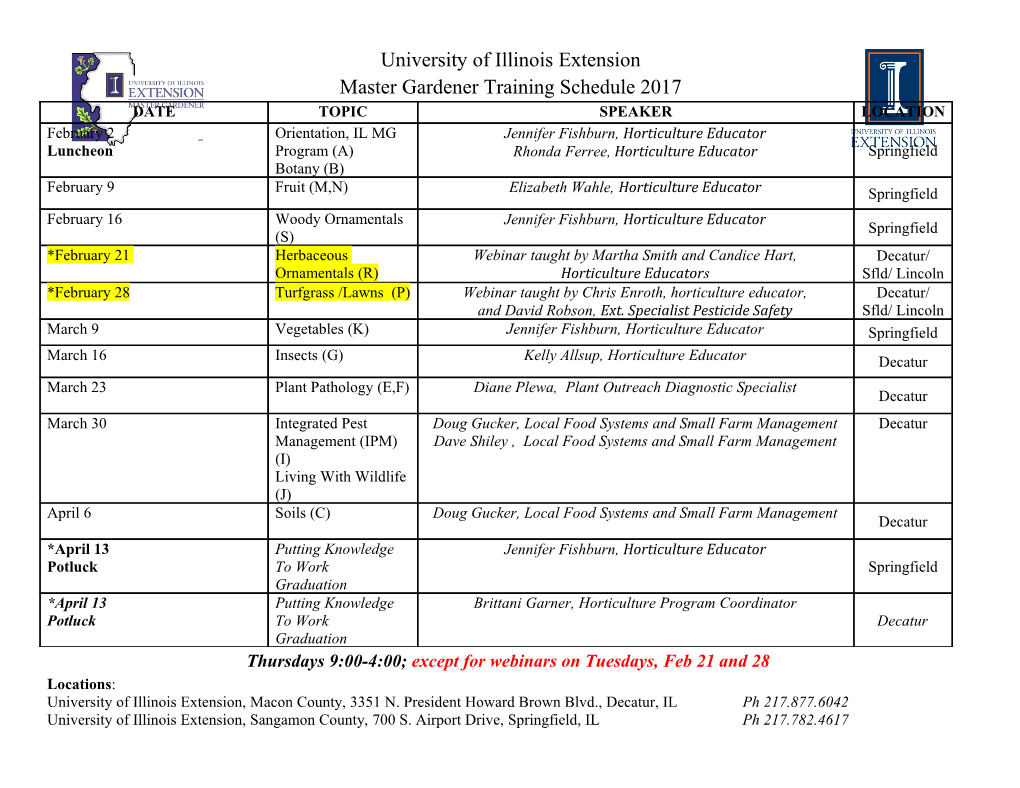
COMMUNICATION A fluorinated detergent for membrane-protein applications Erik Frotscher,[a] Bartholomäus Danielczak,[a] Carolyn Vargas,[a] Annette Meister,[b] Grégory Durand,[c] and Sandro Keller*[a] Abstract: Surfactants carrying fluorocarbon chains hold great prom- broken.[12,13] Moreover, fluorocarbon chains are bulkier than their ise as gentle alternatives to conventional, hydrocarbon-based deter- hydrocarbon counterparts, which prevents them from intruding gents for the solubilization and handling of integral membrane pro- between protein transmembrane domains.[7,12] teins. However, their inertness towards lipid bilayer membranes has The peculiarity of fluorocarbon chains of being both hydrophobic limited the usefulness of fluorinated surfactants in situations where and lipophobic is thought not only to prevent protein denatura- detergent-like activity is required. Here, we show that fluorination per tion and delipidation but also to render fluorinated surfactants se does not preclude detergency, as exemplified by a fluorinated poor solubilizers,[13–18] unless they contain a harsh, ionic head- [19] octyl maltoside derivative dubbed F6OM. This nonionic compound group. Here, we show that, contrary to this assumption, avidly interacts with and completely solubilizes phospholipid vesicles nonionic fluorinated surfactants can be powerful yet mild deter- in a manner reminiscent of conventional detergents without, however, gents. Comparison of two compounds sharing the same fluoro- compromising membrane order at subsolubilizing concentrations. carbon chain but different headgroups reveals that fluorination Owing to this mild and unusual mode of detergency, F6OM outcom- per se does not guarantee lipophobicity. Instead, it is the proper- petes a lipophobic fluorinated surfactant in chaperoning the func- ties of the polar headgroup that, in conjunction with a fluorocar- tional refolding of an integral membrane enzyme by promoting bi- bon chain, govern the detergency—or lack thereof—of fluorinat- layer insertion in the absence of micelles. ed surfactants. While this calls for caution in employing fluori- nated surfactants in experiments where membrane integrity is essential,[20–22] it also paves the way for their judicious use in Most in vitro approaches to membrane proteins rely on deter- biochemical approaches requiring detergent activity without the gents, that is, surface-active compounds that interact with and, need of conventional, hydrocarbon-based detergents. at sufficiently high concentrations, disrupt lipid bilayer mem- branes.[1] Detergent-solubilized proteins can then be purified and subjected to biochemical, biophysical, or structural scrutiny[2] or reconstituted into artificial bilayers.[3,4] However, exposure to detergents often results in the irreversible loss of native protein structure and function due to the disruption of stabilizing interac- tions within the protein or with lipids.[5,6] The recent years have witnessed increasing efforts at developing new amphiphiles that could substitute for conventional detergents in solubilizing mem- brane proteins without compromising their integrity.[7] Notable examples include lipid nanodiscs bounded by scaffold proteins[8] or styrene maleic acid copolymers,[9] amphiphilic polymers known as amphipols,[10] tripod amphiphiles,[11] and fluorinated [12–14] surfactants. The latter retain the most favorable properties Figure 1. Chemical structures of F6OM and F6OPC. of hydrocarbon-based detergents but overcome many of their limitations.[7] By analogy to the poor miscibility of liquid hydro- We first compared self-assembly and membrane solubilization carbons and fluorocarbons, contacts between fluorinated surfac- by 3,3,4,4,5,5,6,6,7,7,8,8,8-tridecafluoro-n-octyl-β-D-maltopyra- tant tails and hydrocarbon moieties of proteins and lipids are noside (F OM) and 3,3,4,4,5,5,6,6,7,7,8,8,8-tridecafluoro- expected to be unfavorable, such that structurally or functionally 6 n-octylphosphocholine (F OPC), both of which carry an octyl important protein/protein and protein/lipid interactions are not 6 chain whose terminal hexyl segment is perfluorinated (Figure 1). Nonionic F6OM self-assembled into elongated, rod-shaped micelles observable by transmission electron microscopy (Fig- [a] E. Frotscher, B. Danielczak, Dr. C. Vargas, Jun. Prof. Dr. S. Keller ure 2a), whereas zwitterionic F6OPC micelles appeared smaller Molecular Biophysics, University of Kaiserslautern and spheroidal, as judged by dynamic light scattering (Fig- Erwin-Schrödinger-Str. 13, 67663 Kaiserslautern, Germany ure S1). This contrasts with an F6OPC analog bearing two more E mail: [email protected] [23] [b] PD Dr. A. Meister CF2 groups, which forms bilayer membranes. Under the con- Center for Structure and Dynamics of Proteins (MZP), Martin Luther ditions used in our experiments, the critical micellar concentra- University Halle–Wittenberg, Biocenter tions (CMCs) of F6OM and F6OPC amounted to 0.7 mM and Weinbergweg 22, 06120 Halle (Saale), Germany 2.9 mM, respectively, as determined by calorimetric demicelliza- [c] Dr. G. Durand [24] 19 Equipe Chimie Bioorganique et Systèmes Amphiphiles, Université tion titrations (Figure S2) and confirmed by F-NMR spec- [16] d’Avignon et des Pays de Vaucluse troscopy (Figure S3). At 25°C, exposure of large unilamellar 33 rue Louis Pasteur, 84000 Avignon, France; vesicles (LUVs) composed of the phospholipid 1-palmitoyl- Institut des Biomolécules Max Mousseron, UMR 5247 CNRS-UM- 2-oleyl-sn-glycero-3-phosphocholine (POPC) to micellar F OM ENSCM 6 15 avenue Charles Flahault, 34093 Montpellier Cedex 05, France resulted in slow but steady solubilization, as indicated by a de- Supporting information for this article is given via a link at the end of the document. COMMUNICATION crease in light scattering intensity (Figure 2b). Solubilization of from the characteristics of a “membranophobic” lipopeptide.[27] 31 19 0.1 mM POPC LUVs required several days at 2 mM F6OM and P- and F-NMR experiments confirmed the concomitant trans- 31 25°C but was greatly accelerated by increasing the surfactant fer of POPC and F6OM from bilayers into micelles. The P concentration to 10 mM (Figure 2b) or raising the temperature to signal was broadened beyond detection in vesicular POPC [28] 60°C (Figure 2c). By contrast, F6OPC did not affect vesicle suspensions because of the slow tumbling of LUVs, but a integrity even at 60°C and a concentration of 20 mM (Figure 2c), sharp isotropic peak appeared upon solubilization by F6OM and in agreement with the poor performance of longer-chain analogs remained constant after complete transfer of POPC into micelles as membrane solubilizers.[15] These differences in solubilizing (Figure 3c,e). In 19F-NMR spectra,[16] the onset of solubilization power rationalize the observation that F6OPC but not F6OM is manifested itself in an upfield shift in the signal of the CF3 group [20] compatible with free-standing lipid bilayers. of F6OM, which continued to change upon variation of the com- position of the mixed micelles by further addition of F6OM (Fig- ure 3d,e). Figure 2. Differential solubilization of lipid vesicles by fluorinated surfactants. a) Negative-stain transmission electron micrograph of 1 mM F6OM. Arrows indicate rod-shaped micelles. b) Kinetics of vesicle solubilization by F6OM at 25°C as reflected in 90° light scattering intensity, I, as a function of time, t. 0.1 mM POPC in the form of LUVs was incubated with 2 mM (black) or 10 mM (gray) F6OM. Horizontal lines represent mean intensities of pure POPC LUVs (dashed) or pure F6OM micelles (dotted) at the respective concentrations. Inset: Intensity-weighted particle size distributions obtained immediately (gray) or 60 h (light gray) after mixing 0.1 mM POPC LUVs with 10 mM F6OM (cf. arrows in main panel). c) Solubilization kinetics at 60°C as reflected in I as a function of t. 0.1 mM POPC LUVs were mixed with 2 mM F6OM (black) or 20 mM F6OPC (gray). A systematic study of the solubilization behavior and the supra- Figure 3. Solubilization of POPC vesicles as a function of F6OM concentration. a) 90° light scattering intensity, I, during solubilization in which POPC LUVs molecular organization of POPC/F6OM mixtures was possible by were challenged with increasing concentrations of F OM. Arrows indicate data recording the intensity of scattered light as a function of the 6 points used for constructing the phase diagram. b) POPC/F OM phase dia- [25] 6 concentrations of both components (Figure 3a). The F6OM gram showing ranges where only bilayers (b), only micelles (m), or both (m+b) concentrations required for initiating and completing solubiliza- exist. Phase boundaries (solid lines with fitting parameters) are linear fits to 31 tion increased linearly with POPC concentration, allowing for the concentration pairs (circles) taken from a). c) P-NMR spectra of 2 mM POPC [26] LUVs mixed with the indicated concentrations of F6OM in 90% H2O/10% D2O. construction of a phase diagram in which a coexistence range 19 d) F-NMR spectra of the same samples focusing on the CF3 resonance of separates two regions where either only bilayer or only micellar F6OM. For clarity, vertical scaling is non-uniform across different F6OM con- structures exist (Figure 3b). As derived from the slopes of the centrations. e) 31P-NMR peak intensities, I, (black) and 19F-NMR peak chemi- phase boundaries, solubilization set in when the molar cal shifts, δp, (gray) of the CF3 group against F6OM concentration. Gray lines indicate phase boundaries predicted by the
Details
-
File Typepdf
-
Upload Time-
-
Content LanguagesEnglish
-
Upload UserAnonymous/Not logged-in
-
File Pages5 Page
-
File Size-