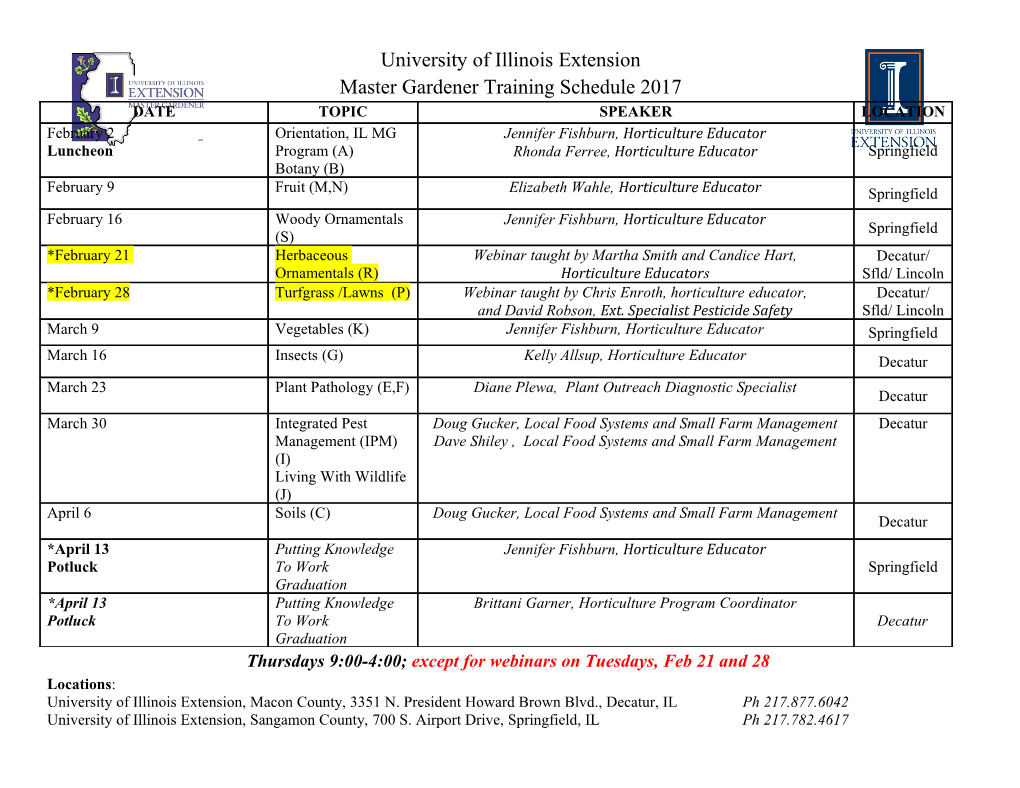
Precession of a planet with a satellite G. Boué, J. Laskar To cite this version: G. Boué, J. Laskar. Precession of a planet with a satellite. Icarus, Elsevier, 2006, 185, pp.312-330. 10.1016/J.ICARUS.2006.07.019. hal-00335321v1 HAL Id: hal-00335321 https://hal.archives-ouvertes.fr/hal-00335321v1 Submitted on 29 Oct 2008 (v1), last revised 29 Oct 2008 (v2) HAL is a multi-disciplinary open access L’archive ouverte pluridisciplinaire HAL, est archive for the deposit and dissemination of sci- destinée au dépôt et à la diffusion de documents entific research documents, whether they are pub- scientifiques de niveau recherche, publiés ou non, lished or not. The documents may come from émanant des établissements d’enseignement et de teaching and research institutions in France or recherche français ou étrangers, des laboratoires abroad, or from public or private research centers. publics ou privés. Precession of a planet with a satellite. G. Bou´eand J. Laskar Astronomie et Syst`emes Dynamiques, IMCCE-CNRS UMR8028, Observatoire de Paris, 77 Av. Denfert-Rochereau, 75014 Paris, France October 29, 2008 Abstract a planet in presence of a distant satellite is well known (see Murray, 1983). In this approximation, the precession The contribution of a satellite in the precession motion of torque, and thus the precession frequency, increases as the axis of an oblate planet has been previously studied in 1/r3 when the distance r of the satellite to the planet de- the approximation of a distant satellite, or in the approxi- creases. It is thus clear that these formulas are no longer mation of a very close satellite. Here we study the general valid for a close satellite. problem for an arbitrary semimajor axis for the satellite, The understanding of the contribution of a close satel- without performing the usual gyroscopic approximation. lite was first motivated by the study of the Martian satel- We present precessional equations valid in a very general lites, Phobos and Deimos. Goldreich (1965) investigated setting, and we demonstrate that this problem, after the first the interaction of a close satellite with a precessing classical expansion of the satellite potential, and averag- planet, and demonstrated that a close satellite will fol- ing over the fast angles, is indeed integrable. We provide low the planet with a nearly constant inclination to the here the complete solution of this problem by quadrature, equator. This work was followed by the contributions of as well as some explicit approximate solutions. We also (Kinoshita, 1993) who analyzed the motion of the Ura- demonstrate that after averaging over the nutation mo- nian satellites under the secular change of the obliquity tion, the pole of the spin axis, the pole of the satellite of the planet, and (Efroimsky, 2004) who consider non orbit, and the pole of the planet orbit remain coplanar uniform precessions. with the total angular momentum and precess uniformly In his beautiful study of the Lunar orbit, (Goldreich, around the total angular momentum. 1966) extended his work to the Sun-Earth-Moon system, Keywords : CELESTIAL MECHANICS, PLANETARY but assumed that the planet orbit is fixed and circular DYNAMICS, ROTATIONAL DYNAMICS, SATEL- orbits for both satellite and planet. This work was ex- LITES DYNAMICS, MOON tended by (Touma and Wisdom, 1994a) using a non aver- aged Hamiltonian, and equations of motion expressed in the mobile frame linked to the planet. 1 Introduction Explicit analytical expressions for the contribution of a close satellite to the precessional motion of a planet We are considering here a relatively simple system com- were derived by Ward (1975), using the equations of Gol- posed of a central star, a planet orbiting the star, and a dreich (1966), with zero eccentricities, zero inclinations, satellite orbiting the planet. We increase the complexity and the gyroscopic approximation (i.e. one assumes that of the problem by considering that our planet is a solid the axis of rotation is the axis of figure of the planet). non-spherical body. The most obvious system of this kind These computations were improved by Tremaine (1991) is the Sun-Earth-Moon system, but some triple star sys- who considered the inclinations, and corrected the mass tems will fit in our study as well. We focus here on the factors of Goldreich (1966). precessional motion of the spin axis of the planet, and in a lesser degree on the precessional motion of the orbital In section 2, we consider the general problem with an plane of the satellite and of the planet. oblate planet and a satellite. Contrarily to many of the The computation of the precession of the spin axis of previous study, we do not make the gyroscopic approxi- mation, thus allowing for an axis of figure different from 1E-mail address: [email protected] the axis of rotation. Nevertheless, in the present work, 1 we simplify the equations of motion by averaging over the rotational motion of the planet, providing some preces- m2 sion (and nutation) equations that can be used in a very r02 r2 general setting. r1 In a second stage (section 3), we derive the secular equa- tions that are obtained by averaging over the orbital mo- O m0 r01 tion of the satellite and the planet, and over the argument m1 of perihelion of the satellite. We obtain a set of secular equations that describe in a closed way, the evolution of the spin of the planet, the orbital plane of the planet, and Figure 1: Jacobi coordinates. the orbital plane of the satellite in a very general setting. We then demonstrate that, quite surprisingly, this dif- ferential system of order 9 with 7 integrals is integrable, problem can be expressed as and can be decomposed as a relative periodic motion (the = H + H + H (1) nutation) and a general precessional motion. The two pe- H N E I riods can be derived by quadratures. We obtain thus some where HN is the Hamiltonian of three mass points, HE general formulas (although not explicit) that provide the describes the free rigid body motion and HI contains the precession formulas for the axis of the planet, in all cases, gravitational interaction between the bulge and the other for a distant or a close satellite, but also in the interme- two mass points. In such a satellite problem, the orbital diate regime where none of the previous approximations Hamiltonian is naturally expressed in Jacobi coordinates, is valid (section 4). In this section, we also demonstrate that after averaging over the nutation motion, the pole r0 1 0 0 u0 of the spin axis, the pole of the satellite orbit, and the r = 1 1 δ δ u (2) 1 − − 1 pole of the planet orbit remain coplanar with the total r 0 1 1 u 2 − 2 angular momentum (section 4.3). This is in some sense a where δ =m /(m + m ). With this choice, r = u is generalization of the Cassini Laws. 2 1 2 0 0 the barycentric position vector of the Sun, r1 the position After some discussion of numerical examples (section vector of the planet-satellite barycenter relative to the 5), in section 6, we proceed to an additional approxima- Sun, and r2 the position vector of the satellite relative to tion that allows to obtain a completely explicit solution of the planet. The symplectic structure is preserved using this problem, for arbitrary values of the eccentricities, in- the conjugate momentum clination, and semi major axis of the planet and satellite, whenever the averaging is possible. We can then compare ˜r0 1 1 1 ˜u0 this approximation with our rigorous expression, and with ˜r1 = 0 1 1 ˜u1 (3) the results of non averaged numerical integrations in dif- ˜r2 0 δ 1 δ ˜u2 − ferent settings. In particular, we use as a test model a ˜u u Lunar motion where the Moon distance to the Earth is where i = mi ˙ i (i = 0, 2) are the barycentric momen- varied from the surface of the Earth to some limit distance tum. In these coordinates, the Newtonian interaction is where, due to the solar perturbation, the Moon escapes, 2 2 ˜r1 ˜r2 µ2β2 m0m1 m0m2 and no longer remains a satellite of the Earth. We make HN (r,˜r)= + G G also comparison of our results with the classical compu- 2β1 2β2 − r2 − r01 − r02 tation in the case of a distant satellite, and the previous (4) expressions of Ward (1975) and Tremaine (1991), for close where µ2 = (m1 +m2),β1 = M12m0/(M12 +m0),β2 = satellites (section 7). G m1m2/(m1 + m2) are the reduced masses with M12 = m1 + m2, and r01, r02 are the modulus of the position vectors r01, r02 from the Sun to the planet and satellite, 2 Fundamental equations expressed as r01 = r1 δ r2; r02 = r1 + (1 δ)r2. (5) We are considering here a three body problem with a cen- − − tral star, an oblate planet, and a satellite orbiting the The Hamiltonian of the free motion of a rigid body is planet, with respective barycentric coordinates u0, u1, u2 1 t 1 and masses m , m , m . The full Hamiltonian of this HE = G − G (6) 0 1 2 2 I 2 where G is the angular momentum of the rigid body and ets are identically equal to zero. B(r,˜r, K, G) is then its inertia tensor. Let (I, J, K) be the principal frame whereI is diagonal ( = diag(A, B, C)). For sake of B(y)= I I clarity, we present here the case of an axisymmetric planet 0 I 0 0 (A = B). The general case can be treated in the same way I −0 0 0 providing some additional averaging, and will be outlined 0 K K − 3 2 in section 2.6.
Details
-
File Typepdf
-
Upload Time-
-
Content LanguagesEnglish
-
Upload UserAnonymous/Not logged-in
-
File Pages24 Page
-
File Size-