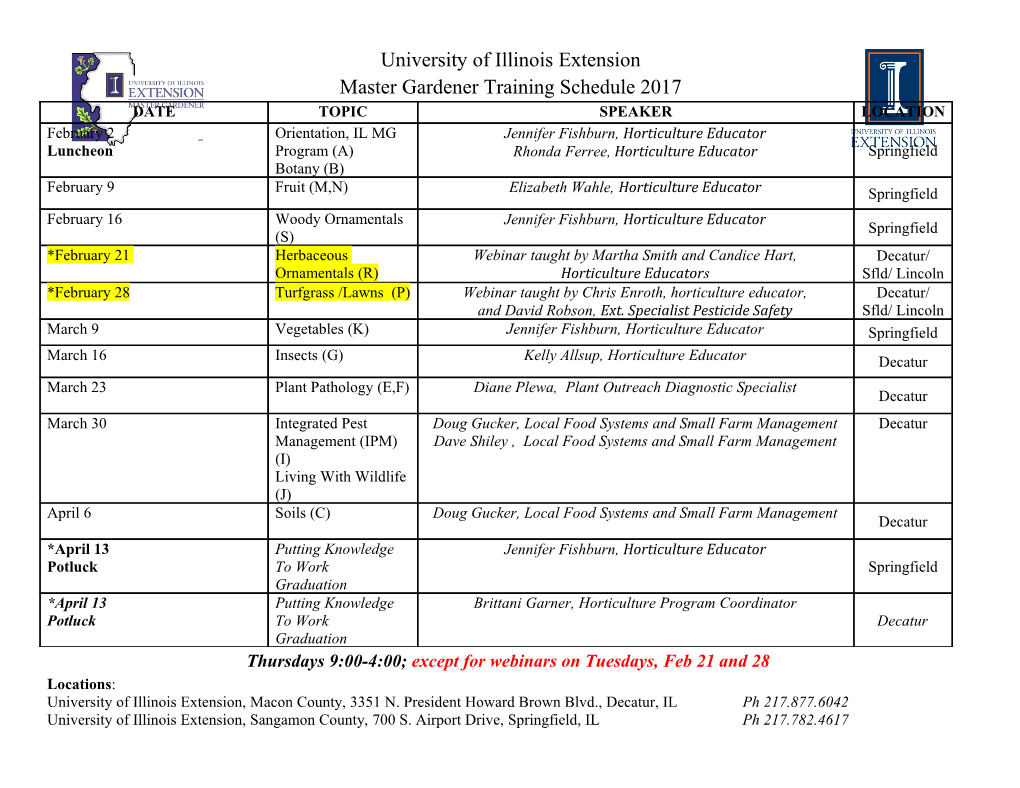
463 Reprinted from the Soil Science Society of America Journal Volume 51, no. 4. July-August 1987 677 South Segos Rd., Madmon, WI 53711 USA Cohesion Development in Disrupted Soils as Affected by Clay and Organic Matter Content and Temperature' w. D. KEMPER, R. C. ROSENAU, AND A. R. DEXTER 2 ABSTRACT OIL STRUCTURE IS DISRUPTED and bonds holding Soils were dispersed and separated into sand, silt, and clay frac- S particles together are broken frequently as soil is tions that were reconstituted to give mixtures of each soil with 5 to frozen at high water contents, wetted quickly, tilled, 40% clay. In the range from 0 to 35% clay, higher clay contents or compacted (e.g., Bullock et al, 1987). As soils dry, resulted in greater stability. Rate of cohesion recovery was over 10 increasing tension or negative pressure in the water times as fast at 90°C as it was at 23°C, showing that the processes (Briggs, 1950) pulls particles together, increasing the Involved are physical-chemical rather than biological. Maximum rates number of contact points at which bonding can take of cohesion recovery occurred at moderate soil water tensions, prob- place in newly disrupted soils. Strengths of aggregates ably because some tension is needed to pull the particles into direct formed from disrupted soils increase with time (Blake contact, but a continuous water phase is also essential to allow dif- and Oilman, 1970; Utomo and Dexter, 1981; Kemper fusion of bonding agents to the contact points. Since diffusion rates and Rosenau, 1984), even when water content re- in water increase 3e0o*, while rate of cohesion recovery increased mains constant, indicating increasing strength of par- 1000% when temperature was raised from 23 to 90°C, other factors, ticle-to-particle bonds. "Soil structure" is dynamic in such as higher Mobilities at higher temperatures of compounds terms of both its geometric and cohesion character- contributing hooding ions to the solution. probably play a role In istics. the rate of cohesion recovery. Recovery of cohesion was more rapid in the soil with organic C contents of 0.004 kg/lig than in the soil The ultimate nature of the particle-to-particle bonds with 0.012 kg/kg. When the organic matter was removed with H 201 is atomic and ionic interactions. However, observa- from the soil with 0.012 kg C/kg, its rate of cohesion recovery in- tions under optical microscopes (50-400 magnifica- creased. Rate of cohesion recovery of this high organic matter soil tion) have shown us that as recently disturbed sandy was also increased by aging it at 0.1 kg HP/kg soil compared to soil dries, the retreating menisci pull small suspended 0.2 kg/kg. A possible explanation is that organic coatings, tending mineral particles toward the points of contact between to prevent direct contact and bonding of adjacent projections of min- sand grains, forming additional contacts that bond the eral surfaces, are forced away from contact points by extremely strong sand grains together. Methods for estimating strengths forces that pull the adjacent minerals together when soil water ten- of sandy materials bonded by clays have been pro- sions are high. When the higher organic matter soil had been con- posed by Ingles (1962) and Mullins and Panayioto- solidated by air-drying and rehydrated, its rate of cohesion recovery poulos (1984). These observations and the general was just as rapid as that of the soil with low organic matter. positive correlation between clay content and aggre- Additional Index Words: diffusion, mineral bonding, interparticle gate stability in arid and semiarid soils (Kemper and contact, water tension. Koch, 1966) indicate that clay plays a major role in the cohesion of larger particles. The following study Kemper, W.D., R.C. Rosenau, and A.R. Dexter. 1987. Cohesion was designed to further establish and define the role development in disrupted soils as affected by clay and organic mat- of clay and related factors in cohesion. ter content and temperature. Soil Sci. Soc. Am. J. 51:860-867. PROCEDURE ' Contribution from USDA-ARS, Snake River Conservation Re- search Center, Kimberly, ID 83341. Received 12 May 1986. Percentages of sand, silt, and clay; organic C content; and = Soil Scientist, Agricultural Research Technician, and Consultant pH values are given, with locations of origin of the soils (at SRCRC in July to August 1985, from the Waite Inst., Univ. of used in this study, in Table 1. Mineralogic descriptions of Adelaide), respectively. the clays in these soils indicate that illite is the dominant 860 KEMPER ET AL.: COHESION DEVELOPMENT IN DISRUPTED SOILS 861 type of clay in all three of them with smaller amounts of RESULTS, DISCUSSION, AND smectite, vermiculite, and kaolinite also present. SUPPLEMENTARY OBSERVATIONS These soils were dispersed sonically, and three portions of the sediment containing primarily sand, silt, and day, Moduli of rupture (MR) of cores made of separates respectively, were separated by sedimentation. Particle size from each of the three soils containing 0.2 kg H10/kg distribution in these portions was determined by the hy- soil are ahown in Fig. 1. In each soil the MR increased drometer method (Gee and Bauder, 1986), and the mineral as clay content increased. Higher clay contents pro- portions were air-dried after allowing them to settle for 7 d, vide more opportunities for interparticle contact and and decanting the supernatant liquid. Using the measured also adsorb more water, causing higher tensions in soil particle size distributions in the dominantly sand, silt, and water at a specific water content (0.2 kg/kg in this clay portions of the soil, the amounts of each portion re- quired to provide 100 g each of soil materials containing 5, study). The MR also increased with time, indicating 10, 15, 20, 25, 30, 35, and 40% clay was calculated. Ratios that in these moist soils, interparticle bonds strengthen of silt/sand were kept within 10% of their values in the orig- with time. inal soil sample. The dried materials were then crushed, Increased strength due to a constituent such as clay mixed in the calculated portions, and 100 g of soil was wet- helps aggregates resist breakdown when they are sub- ted with 20 g of water. This wetted soil was then forced jected to shearing forces by tillage, freezing, fast wet- through a 2-mm sieve forming extruded "aggregates." These ting, compaction, etc. Consequently, the strengthening aggregates were stable while there was still tension in the constituent helps maintain a more open structure with water holding the particles together. However, if they were fewer particle-to-particle contacts than would have de- immediately immersed in water, which relieved that ten- veloped if the structure had disintegrated and the soil sion, the aggregates disintegrated. Aggregates of each soil containing 5, 15, 25, 35, and 40% particles had been pulled into denser packing by forces clay were stored in separate sealable jars. Two 3.6-g samples due to gravity and water tension (e.g., Kemper et al., were taken immediately from each jar and exposed to an air 1975). As Dowdy and Larsen (1971) pointed out, stream carrying water vapor until they contained a total of strength of the soil is limited by the number and 0.9 g of water per sample of aggregates. Their wet stabilities strength of bonds between the aggregates_ Conse- were then determined by placing them in sieves with 0.26- quently, the strength of soils, as measured on cores in mm openings, immersing the aggregates in distilled water, this study or on other macroscopic soil units such as oscillating the screens up and down for 3 min, and deter- crusts, is highly dependent on previous disruptions mining the portion of the particles <0.26 mm in diameter, and time and water content following disruption. These which remained in aggregates >0.26 mm in diameter. (Os- cillations were sinusoidal with amplitude of 13 mm and a confounding factors often result in poor or negative frequency of 35 oscillations/min. See Kemper and Rosenau, correlations between strength (modulus of rupture, pe- 1986, for further procedural details.) Time from extrusion netrability, etc.) measured on macroscopic soil units to wet sieving was about 20 min. Other sets of duplicate samples were taken from the sealed jars and wet sieved after FROM they had aged for 4 h, 1 d, 5 d, and 30 d following extrusion. 14 MUMS CLAY While aging, the sealed glass jars were stored in a dark cab- 12 inet at about 22°C. Water contents of aggregates remaining 10 30 days in the sealed jars after 30 d were the same as those 20 min e 0 after extrusion. 6 4 minutes alter Moduli of rupture (MR) were determined on cores of soil --",„ 4 packing In aynnaere 2.5 cm in diameter and about 5 cm long by laying the cores 2 between two flat boards and increasing the force pushing "1 0 those boards together until the cores ruptured (Kirkham et •n. 10 20 30 40 al., 1959). The cores were formed by pouring the extruded 0 F ROM aggregates into brass cylinders containing an Al foil inner =.`: 14 PORTNEUF SILT LOAM liner and compacting when the cylinders were about 1/4, 1/2, la 12 3/4, and 4/4 full with a force of 80 kPa. M 10 I- After packing, the inner liner and soil core were pulled Q. 8 out of the brass cylinder and the foil was peeled off the soil cr• 6 core. Moduli of rupture were determined on duplicate cores 4 made of materials from each soil 4 min after packing for q 2 samples with clay contents of 5, 15, 25, 35, and 40%.
Details
-
File Typepdf
-
Upload Time-
-
Content LanguagesEnglish
-
Upload UserAnonymous/Not logged-in
-
File Pages7 Page
-
File Size-