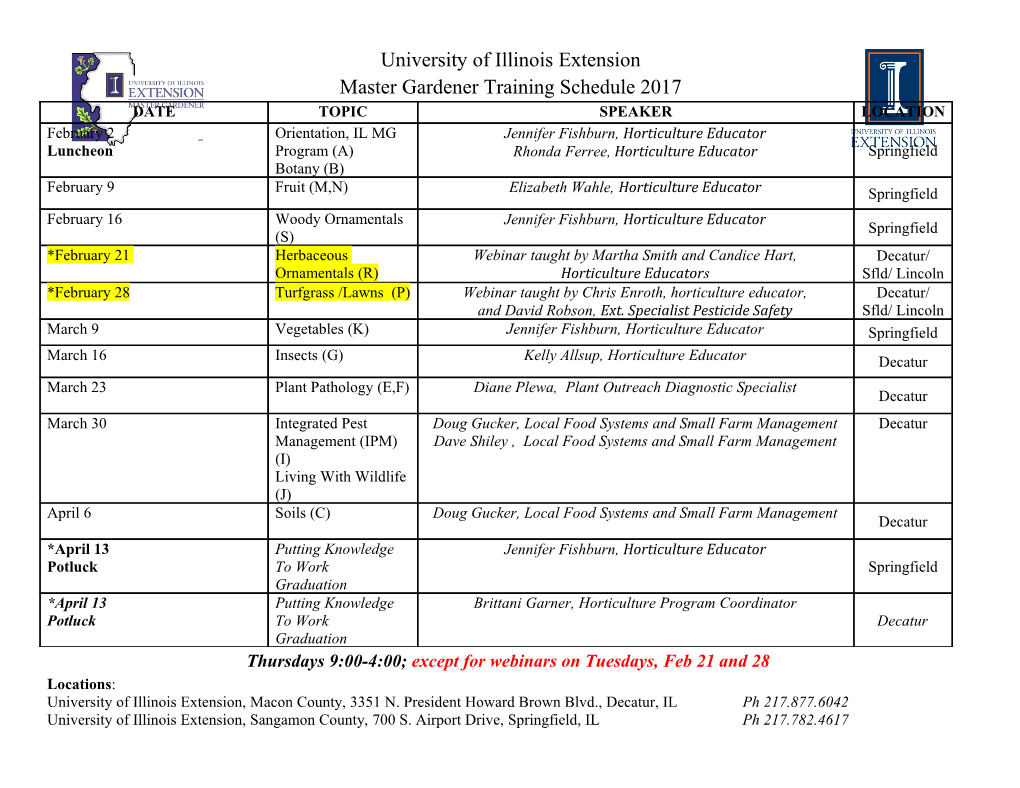
CHAPTER 2: IN SITU OXYGEN ISOTOPE GEOCHEMISTRY BY ION MICROPROBE John W. Valley & Noriko T. Kita, WiscSIMS Laboratory Department of Geology and Geophysics University of Wisconsin Madison, WI 53706 U.S.A. [email protected] INTRODUCTION included in these reviews. However, the High precision and accuracy with in situ conventional analytical techniques employed in analysis has been a “holy grail” for generations of these studies require homogenization of bulk stable isotope geochemists who employed a range samples that are larger than the scale of zonation in of strategies for separation of heterogeneous a wide range of samples. Important information has samples and for interpretation of data from mixed been lost by analysis of powders. samples. However, in situ techniques have in the The ion microprobe’s ability to analyze past suffered from relatively poor precision and isotope ratios with high precision and accuracy accuracy. Recent advances by ion microprobe have from micrometre-scale spots in natural samples (as improved precision and accuracy, and make these well as experimental products) in situ from a time-consuming, uncertain approaches unnecessary. microscope slide reduces sample size by factors of In situ analyses can be correlated with textures and ten thousand to one billion, and allows isotopic data imaging, offering the promise of new and to be correlated with other geochemical information fundamental information about patterns of isotope in spatial context with textures and imaging (Fig. distribution. 2-1). The accuracy of these data now approaches The importance of stable isotope that of conventional techniques that require far geochemistry is well established in many disciplines larger samples. These advantages are revolution- (see Clark and Fritz 1997, Griffiths 1998, Kendall izing stable isotope geochemistry, just as the & McDonnell 1998, Criss 1999, Ambrose & electron microprobe revolutionized in situ chemical Katzenberg 2000, Valley and Cole 2001, Unkovich analysis and the SHRIMP (ion probe) et al. 2001, Hoefs 2004, De Groot 2004, Johnson et revolutionized zircon geochronology (Ireland 1995, al. 2004, Sharp 2007). Many seminal studies are Hinton et al. 1995, Ireland & Williams 2003). 18 FIG. 2-1. Correlated ion microprobe analysis of δ O, U-Pb, and trace elements in zircon. Left: CL image of a detrital zircon from the Jack Hills metaconglomerate, Australia, showing a concentrically zoned igneous core dated at 4.1 Ga and thin overgrowths that are younger than 3.7 Ga. Analysis pit locations are shown for δ18O, U-Pb, and REEs. Scale bar = 100 μm. Right: Cartoon of a zoned detrital zircon showing a strategy for three successive analyses from a domain <20 μm in diameter: U-Pb (surface 1), δ18O, and trace elements (surface 2, after repolishing). Note that zoning is finer than spot size; sub-μm analysis will be discussed below (from Cavosie et al. 2006). Mineralogical Association of Canada Short Course 41, Toronto, May 2009, p. 19-63 19 J.W. VALLEY & N.T. KITA This chapter discusses procedures for high gun); ±2‰ (IMS4f, high mass resolution); ±1.0‰ precision and accuracy of ion microprobe analysis (IMS4f, high energy offset); ± 0.6‰ (IMS1270, of oxygen isotope ratios, and reviews recent studies multi-collector); ± 0.3‰ (IMS1280) (Giletti et al. that have applied these techniques to Earth Science. 1978, McKeegan 1987, Valley & Graham 1991, It is beyond the scope of this chapter to discuss all Hervig et al. 1992, Riciputi & Patterson 1994, of the earlier pioneering studies; additional Cavosie et al. 2005, Kelly et al. 2007, Kita et al. references can be found in papers that are cited. 2009). Precision is emphasized in this section Strategies for ion microprobe analysis vary before discussion of accuracy. Accuracy equal to according to trade-offs in instrument tuning, sample precision can be attained in many samples by preparation, and analysis. Because of improved data detailed monitoring of instrumental conditions, quality at smaller spot sizes, applications discussed careful sample preparation, and use of appropriate here emphasize results from the newest large- standards. While ion microprobe precision for δ18O radius, multi-collector ion microprobe, IMS1280. has improved by an order of magnitude every 15 These studies provide detailed records of zonation years since the first analyses were published (Giletti in samples including diagenetic cements, et al. 1978), and sample sizes have been reduced, speleothems, foraminifera, otoliths, gems, zircon this progress is reaching a plateau. Recent studies grains, and meteorites, which were previously come close to the physical limit imposed by the inaccessible to analysis. In many cases, number of atoms in spot sizes of 10 μm and below understanding the true scale and patterns of isotope (Page et al. 2007a). variation provides new insight for processes as The following figures present large data sets varied as diagenesis, paleoclimate, biomineraliz- for δ18O from 10, 3, and <1-μm diameter spots, and ation, metamorphism, magma genesis, crustal δ17O from 15, 2, and 1 μm spots. A number of evolution, and formation of the Solar System. factors affect data quality. In general, there is a trade-off of precision, which may be limited by the HIGH PRECISION IN SITU ANALYSIS OF number of ions counted, and spot size. OXYGEN ISOTOPE RATIOS Figure 2-3 shows δ18O values from 3.5-minute 18 Analytical precision for analysis of δ O by analyses of 10 μm diameter spots in quartz. Over ion microprobe has improved steadily over the past 650 spot analyses were made in 48 hours from 12 three decades due to development of new different sample mounts. A quartz standard was instrumentation and refinements in technique (Fig. analyzed four times every 10-20 sample spots. The 2-2): ±20‰ (2SD, IMS3f without electron-flood sample data vary by over 40‰, revealing exciting trends in magmatic hydrothermal systems, but the 20 standard data are constant with precision of ±0.3‰ (2 SD, standard deviation; ±0.02‰, 2 SE, standard 3f 4f 1280 error, N = 173). The repeated analysis of an 10 30mm 10mm 20 min. 3.5 min. appropriate standard and a well-prepared sample O ‰) 18 ensures that accuracy matches spot-to-spot d precision. 0 Careful tuning of the instrument, sample preparation, and standardization are critical for 1270 -10 obtaining results such as shown in Figure 2-3. 20mm Pertinent analytical details will be discussed in ± 2 Std. Dev. ( ± 2 Std. Dev. 5 min. subsequent sections of this paper. Attention to detail -20 pays off and it is important to note that not all ion microprobe data are of comparable quality. 1980 1990 2000 Procedures for reporting accuracy and precision Year of publication vary, and the interested readers may want to evaluate precision and accuracy for themselves FIG. 2-2. The precision of ion microprobe analyses for δ18O has improved from ±20‰ in 1978 to ±0.3‰ (2 from the published tables of standard data. In SD) today due to the development of new instruments general, this requires that standard analyses bracket (IMS3f to IMS1280) and refinements in technique (see samples and that data be published in chronological text). At the same time, accuracy, spot size, and speed order of analysis. of analysis have also improved. 20 IN SITU OXYGEN ISOTOPE GEOCHEMISTRY BY ION MICROPROBE 18 FIG. 2-3. Results from 48 hours of analysis of δ O in quartz from 12 sample mounts by IMS1280 at WiscSIMS. Data are from Rusk et al. (2007). The 658 spots analyzed include 173 from the quartz standard, UWQ-1 (solid dots). Grains of standard were cast in each mount. (a) Average value with 2 SD error bars for each group of 4 bracketing standard analyses. Arrows indicate sample changes. The spot-to-spot precision for each group of 8 standard analyses varies from 0.14 to 0.38 and averages 0.29‰ (2 SD; ±0.10‰ 2SE). (b) The 485 analyses of hydrothermal quartz samples (open circles) vary by 40‰ (from Kita et al. 2009). Analysis of δ17O in oxygen three-isotope WiscSIMS, typical values for the 2 SD reproducib- studies is more challenging because of the ~5x ility of δ18O for multiple analyses of a homogeneous lower natural abundance of 17O than 18O. Figure 2-4 material are ± 0.3‰ at 10 μm (Fig. 2-3), ± 0.7‰ at shows δ18O and δ17O in an olivine standard, and 3 μm (Fig. 2-5), and ± 2‰ at <1 μm (Fig. 2-6). samples of meteoritic olivine measured from 15 μm spots using an IMS1280 and three Faraday Cup MULTI-COLLECTOR ION MICROPROBES detectors (Kita et al. 2008). Sample analyses are A number of ion microprobe instruments have bracketed by analyses of standard in the same been used for stable isotope analysis. Early workers mount. The average values of precision for each used single-collector (detector) instruments such as group of eight bracketing standard analyses are the IMS4f. More recently, multicollector 0.31‰ and 0.37‰ (2 SD) for δ18O and δ17O, instruments have demonstrated key advantages. At respectively (Kita et al. 2009). present, the IMS1270/1280 series has proven best Spot size is variable for ion microprobe for the high precision needed for natural isotope analysis. The Cs beam, used for oxygen isotopes, abundances and sub-permil accuracy, but the can be focused to a diameter of 250 nm on the multicollector nanoSIMS and SHRIMP II have also IMS1280 instrument, and 50 nm on the nanoSIMS. been employed, as have single channel instruments. However, the primary beam current and the The first commercial ion microprobe multi- resulting signal varies with spot size, and the beam collector system was installed on an IMS1270 in is generally defocused to a larger diameter in order 1998. This development led to major improvements to generate more secondary ions for analysis. At in stable isotope performance, analogous to the 21 J.W.
Details
-
File Typepdf
-
Upload Time-
-
Content LanguagesEnglish
-
Upload UserAnonymous/Not logged-in
-
File Pages45 Page
-
File Size-