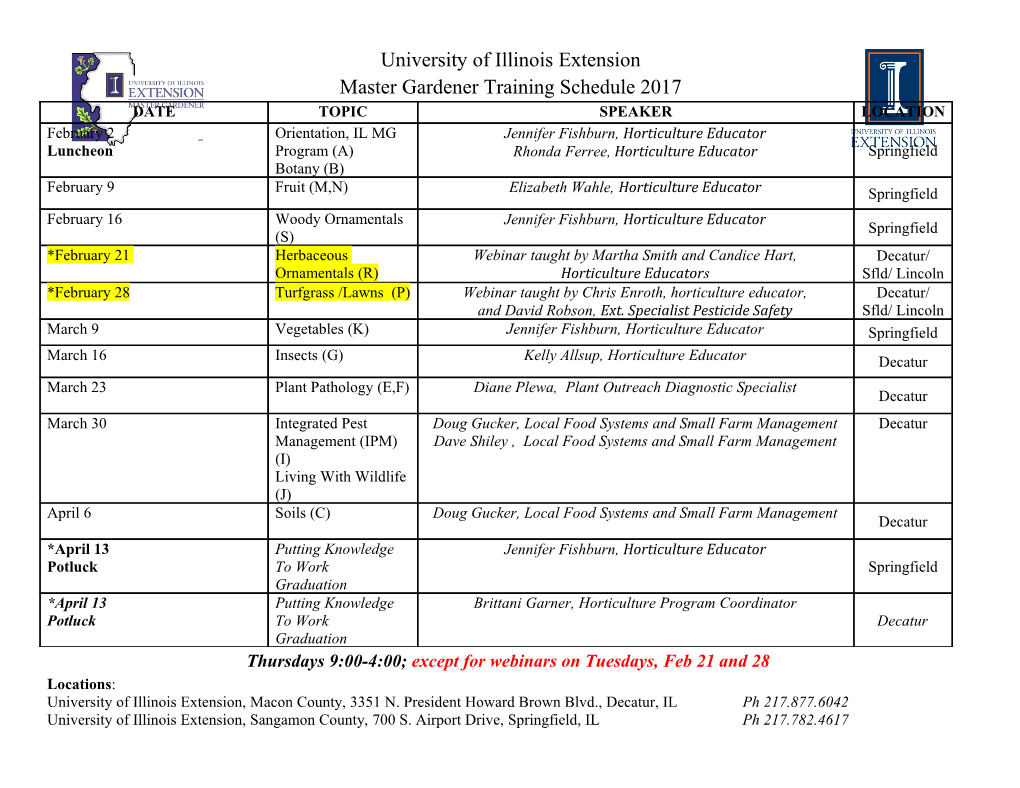
1 Revision 1 2 Uranium scavenging 3 during mineral replacement reactions 4 5 Kan Li,1 Allan Pring,2,4 Barbara Etschmann,1,2,7 Edeltraud Macmillan,3 Yung 6 Ngothai,1 Brian O’Neill,1 Anthony Hooker,1,5 Fred Mosselmans,6 and 7 Joël Brugger2,7* 8 9 1School of Chemical Engineering and 3School of Earth and Environmental Sciences, 10 The University of Adelaide, 5000, South Australia, Australia 11 2Division of Mineralogy, South Australian Museum, North Terrace, 5000, Adelaide, 12 South Australia, Australia 13 4School of Chemical and Physical Sciences, Flinders University, 5001, South 14 Australia, Australia 15 5Radiation Health, Radiation Protection Branch, Environment Protection Authority, 16 Victoria SQ, 5000, Adelaide, South Australia, Australia 17 6Diamond Light Source Ltd, Diamond House, Harwell Science and Innovation 18 Campus, Didcot, Oxfordshire, UK, OX11, 0DE 19 7School of Earth, Atmosphere and Environment, Monash University, 3800, Clayton, 20 Victoria, Australia 21 22 *Corresponding author: [email protected] 23 24 1 25 26 Abstract 27 Interface coupled dissolution-reprecipitation reactions (ICDR) are a common 28 feature of fluid-rock interaction during crustal fluid flow. We tested the hypothesis 29 that ICDR reactions can play a key role in scavenging minor elements by exploring 30 the fate of U during the experimental sulfidation of hematite to chalcopyrite under 31 hydrothermal conditions (220-300 ˚C). The experiments where U was added, either 32 as solid UO2+x(s) or as a soluble uranyl complex, differed from the U-free 33 experiments in that pyrite precipitated initially, before the onset of chalcopyrite 34 precipitation. In addition, in UO2+x(s)-bearing experiments, enhanced hematite 35 dissolution led to increased porosity and precipitation of pyrite+magnetite within the 36 hematite core, whereas in uranyl nitrate bearing experiments, abundant pyrite formed 37 initially, before being replaced by chalcopyrite. Uranium scavenging was mainly 38 associated with the early reaction stage (pyrite precipitation), resulting in a thin U- 39 rich line marking the original hematite grain surface. This ‘line’ consists of 40 nanocrystals of UO2+x(s), based on chemical mapping and XANES spectroscopy. 41 This study shows that the presence of minor components can affect the pathway of 42 ICDR reactions. Reactions between U- and Cu-bearing fluids and hematite can 43 explain the Cu-U association prominent in some iron oxide-copper-gold (IOCG) 44 deposits. 45 46 Keywords: uranium, scavenging, IOCG deposits, experiment, sulfidation reaction, 47 interface coupled dissolution-reprecipitation reactions. 2 48 Introduction 49 Iron oxide-copper-gold (IOCG) deposits, such as Olympic Dam (OD) and 50 Prominent Hill in South Australia, are important sources of copper, uranium, gold, 51 and silver. Uranium is always enriched in South Australian IOCG ores, acting as a 52 penalty element or a resource depending on grade and distribution. OD is 53 characterized by a relatively oxidized mineral assemblage; magnetite-pyrite 54 precipitated at the periphery of the deposit, and hematite dominates in the ores, with 55 a barren hematite body forming the core of the deposit. Copper minerals show an 56 increase in Cu:S ratio towards the core of the deposit: chalcopyrite dominates at the 57 periphery, followed by bornite and finally chalcocite. Bastrakov et al. (2007) 58 suggested that the mineralogical zoning at OD and the stable isotopic (O, S) 59 composition of ore minerals is consistent with a two stage model, whereby a hot, 60 highly saline fluid (>30 wt% NaCl; > 400 ˚C) sourced from a granitic magma 61 formed a low-grade, relatively reduced, magnetite-chalcopyrite orebody, which was 62 later remobilized and upgraded by interaction with meteoric, oxidized fluids. 63 Although OD is the World’s largest U deposit in terms of metal endowment, 64 no specific study on the genesis of U mineralization has been conducted (Cuney, 65 2009). Leaching of U from the wall rocks and reaction of the U-bearing 66 hydrothermal fluids with the Fe-oxide and Cu-sulfide mineralization is assumed to 67 be responsible for U enrichment in some IOCG deposits by a factor of 10 to 40 68 relative to the fresh host rocks (Hitzman et al., 1992; Hitzman and Valenta, 2005). In 69 contrast to the Cu-bearing minerals, the U-bearing minerals do not show a clear 70 zonation pattern at OD. Uranium minerals occur throughout the Cu-enriched zone; 71 uraninite is associated mainly with Cu-Fe sulfides and hematite; brannerite and 72 coffinite are associated mainly with sulfides, quartz, and sericite (Cuney, 2009). 3 73 The importance of fluid-mediated interface coupled dissolution reprecipitation 74 (ICDR) reactions in geological processes has been emphasized over the past decade 75 (Putnis, 2009; Putnis and John, 2010). These reactions are key to understanding ore 76 formation processes, grade control, and the evolution of ore textures, e.g., alteration 77 of pentlandite in the cementation zone (Tenailleau et al., 2006); replacement of 78 magnetite and pyrrhotite by Fe-sulfides (Qian et al., 2010); hematite by chalcopyrite 79 and bornite (Zhao et al., 2014); scavenging of bismuth and gold during ICDR 80 reactions (Tooth et al., 2011); or reactions involving Au-Ag-tellurides (Okrugin et al., 81 2014; Zhao et al., 2009; 2013); or Fe-Ti oxides (Janssen et al. 2010, 2011). 82 Replacement reactions are prominent in IOCG deposits, involved in local and 83 regional alteration, and in upgrading of the Cu and U ores via reaction with 84 preexisting iron-oxide ores. 85 The aim of this study is to test the hypothesis that U is scavenged from the 86 hydrothermal fluids during ICDR reactions, helping to elucidate the Cu-U 87 association in IOCG deposits. We used the replacement of hematite by chalcopyrite 88 under hydrothermal conditions as a model reaction, as this reaction was recently 89 studied by Zhao et al. (2014). Understanding the processes that scavenge U during 90 fluid-rock interaction is key not only for understanding the distribution of U in these 91 deposits, but also for addressing the potential effect of ICDR reactions in promoting 92 or inhibiting metal recovery during ore processing via hydrometallurgical or in-situ 93 leaching methods. 94 Starting materials 95 Hematite sulfidation experiments in the presence of sulfur were conducted 96 following the method of Zhao et al. (2014), by reacting hematite, copper(I) chloride, 4 o 97 and thioacetamide in the presence of water at 220 and 300 C, Psat. Analytical grade 98 CuCl (Hopkin and Williams Ltd.) purified using the procedure of Keller et al. 99 (1946), and thioacetamide (CH3CSNH2) (Scharlau Chemie SA) were used in the 100 experiments. Thioacetamide is stable at room temperature, but it decomposes above o 101 100 C and releases H2S. The solution pH at the reaction temperature was buffered - 102 by the HS /H2S(aq) buffer. To better constrain the pH, we added sodium acetate - 103 (0.14 m) and acetic acid (0.059 m) to shift the HS /H2S(aq) buffer, as well as 1 molal 104 NaCl to prevent disproportionation of Cu(I) complexes (Zhao et al., 2014). Using the 105 HCh thermodynamic equilibrium program (Shavarov, 1999), a pH300˚C of 5.89 was 106 calculated for the system that consisted of 0.0025 moles H2S and HN3, 107 0.000295 mole CH3COOH, 0.0007 mole CH3COO Na, 10 mg hematite and 12.5 mg 108 CuCl in 5 ml H2O. Uranium was introduced as uraninite (see below) or uranyl nitrate 109 (H.B. Selby and Co. Pty. Ltd). 110 Hematite (SA Museum collection number G6983) was used as the starting 111 material. The identification and composition of this mineral was checked by powder 112 X-ray diffraction (XRD; Huber Guinier Imaging Plate G670 with Co Kα1 radiation) 113 and by electron probe microanalysis (Cameca SX-52 operated at 20 kV, 20 nA). The 114 following probe standards were used: Fe2O3 and Fe3O4 (Fe); Mg3Al2(SiO4)3 (Si, Al 115 and Mg); Ca5(PO4)3F (P); MnSiO3 (Mn) and V metal (V). The analysis of hematite 116 (10 points) showed that the starting material was pure (>99.5% hematite 117 components), although some crystals contained micro-inclusions of silica (apparent 118 SiO2 contents up to 3.0 wt%). Crystals were washed ultrasonically, ground and 119 sieved into 125 to 150 µm size fractions. 5 120 Synthetic uraninite was used for the experiments. It was produced by the 121 reduction of high purity UO2(NO3)2 by Zn metal as follows: UO2(NO3)2 was 122 dissolved in 1 molar HCl; O2(aq) was removed by bubbling a 99.5% N2 and 0.5% H2 123 gas mixture through the solution, which was then sealed into Teflon-lined 124 autoclaves. The autoclaves were kept at 150 oC for 3 days. After cooling, the 125 products were washed in 10 molar HCl several times until no further bubbles were 126 released in order to remove Zn and U(VI) impurities. Finally, the products were 127 washed in Milli-Q water (conductivity of 18 MΩ cm-1, Direct-Q3 system, Millipore 128 Corp) and acetone. The synthetic uraninite has a unit cell size a = 5.4105(2) Å (V = 129 158.38(2) Å3), as refined using TOPAS (Bruker, 2005). This unit cell dimension is 130 typical for uraninite found in hydrothermal environments (Fritsche and Dahlkamp, 131 1997), and corresponds to a uraninite composition UO2.56 (Grønvold, 1955; Singh et 132 al., 2011). 133 Experiments and characterization 134 Hematite (10 mg), CuCl(s) (12.3 mg), CH3CSNH2 (187.5 mg), and either 135 uraninite (80 mg) or UO2(NO3)2(s) (116.7 mg, corresponding to ~3,000 ppm U in 136 solution), were loaded into Ti-autoclaves with an 8 ml internal volume in an argon- 137 filled glove box together with 5 ml of a 0.2 m acetate buffered solution.
Details
-
File Typepdf
-
Upload Time-
-
Content LanguagesEnglish
-
Upload UserAnonymous/Not logged-in
-
File Pages29 Page
-
File Size-