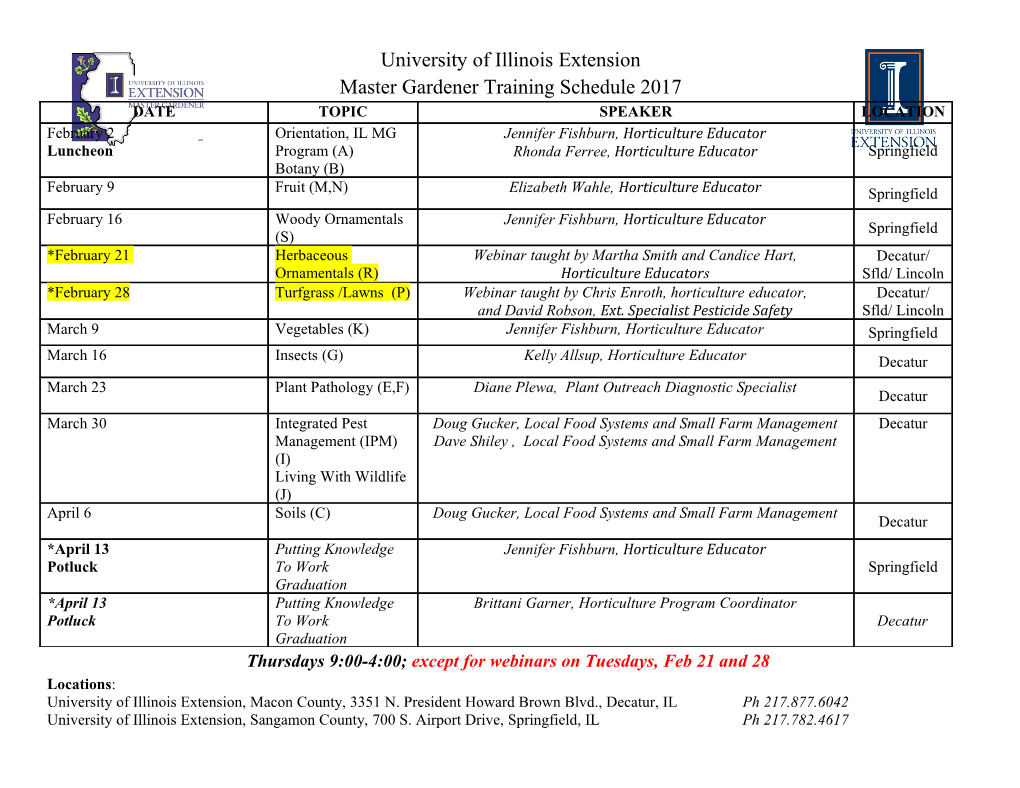
S. Roke: Nonlinear spectroscopy of bio-interfaces Sylvie Roke Max Planck Institute for Intelligent Systems (formerly Max Planck Institute for Metals Research), Stuttgart, Germany Nonlinear spectroscopy of bio-interfaces . ion ut ib str In the past five years the Max Planck Research Group for di Nonlinear spectroscopy of bio-interfaces has worked at the intersection of surface science, nonlinear optics and soft onic tr and biological matter. For hard matter there is molecular level understanding of the surface region. This has enabled elec r researchers to develop the highly complex structures that fo t are nowadays found in (e.g.) computers, and mobile o phones. Although biological and soft systems (such as liq- N uid droplets, nanoparticles, liposomes, and viruses) are ubi- s. e t i quitous in our daily lives, our understanding is often limited ts to macroscopic theories. Consequently, the level of control of soft and biological matter is largely empirical and far be- ane Fig. 1. Unequal numbers in bulk and surface. Illustration of the num- tr hind that of hard matter. To initiate a change, we have in the in past period worked on three main themes: (i) The investiga- ber of atoms situated at the interface and in the bulk of spherical parti- r cles with radius R. o tion of structure and properties of biologically and medi- t cally relevant interfaces (supported lipid bilayers, biopoly- ne r e mer interfaces, water, protein-surface interactions). (ii) their exceptional fluorescence. A small change in the interfa- t n Development of nonlinear light scattering techniques as cial structure, or an increase in interfacial material (brought ni probes for molecular structure at the interfaces of small par- about by a reduction of size) can dramatically alter the color ei s ticles. (iii) The study of molecular interfacial structure, ki- and intensity of the fluorescent light. The stability of emul- ru netics and dynamics of water, surfactants, phospholipids, sions is determined by the interactions (van der Waals forces, fo sugars and peptides on small objects (nano-droplets, vesi- electrostatic interaction, etc.) that occur in the interfacial re- t o cles) in suspensions. The result of our work on these themes gion. Surface modified beads phase separate readily upon N is summarized for this special issue article. the addition of certain proteins and enzymes and can thus be used as sensitive biochemical sensors. Although we some- de Keywords: Non-linear spectroscopy; Interfaces; Liquids; times have a clear picture of what happens on the macro- r. Emulsions; Light scattering and microscopic length and time scales, we do not (yet) un- ijm derstand the underlying molecular picture. ww. In this review of the research of the Max-Planck Research 1. Introducing Group “Nonlinear spectroscopy of bio-interfaces”, we start with a description of nonlinear optical surface spectroscopy. yw Interfaces play a key role in many processes. They play a We then explain some limitations of the method and the steps we have taken towards improvement. Using those improve- man regulating role in transport and structural phenomena in bi- r e ological cells, they can determine the chemistry and phase ments we have started to study biologically relevant surfaces G behavior of colloidal systems, they are important for the such as poly-sugar films and biological samples consisting of mechanical properties of amorphous solids and they can de- self assembled monolayers and proteins. We then move on to nich, a description of developments in the field of nonlinear light u termine the electrical properties of electronic devices. A M large portion of natural matter exists on a micro- and nano- scattering (NLS). We summarize the theoretical advance- scopic scale. Living cells, organelles, colloidal systems, ments made by our research group and show how surface–sol- lag, r emulsion, micelles, and many other systems are composed vent effects can be important. Finally, we describe first results Ve of (sub)micron sized parts. For such systems, the relative obtained from probing the liquid/liquid interface of nano- r e interfacial area – and consequently the importance of inter- scopic oil droplets in water. This article is meant as an over- s face atoms and molecules – increases. view of the activities at the MPI for Metals Research. For an complete referencing we refer to the original publications. H The relative number of atoms and molecules present on l r a the interface of a material increases dramatically when the C material is reduced in size (see Fig. 1). For micro- and nano- 2. Probing planar substrates 11 scopic systems it is well-known that the interfacial region is a 20 dominating factor in determining the physical and chemical 2.1. Nonlinear optical spectroscopy – an interface properties of a material. For such nanoscopic systems it is specific tool known that a change in the interfacial properties results in profound changes in the physical and/or chemical properties. During recent decades several successful attempts have For example, semiconductor quantumdots are known for been made to study planar interfaces on the molecular level 906 Int. J. Mat. Res. (formerly Z. Metallkd.) 102 (2011) 7 FFeature S. Roke: Nonlinear spectroscopy of bio-interfaces and under ambient conditions. One particularly successful 3. Poly-sugars and proteins at interfaces development is based on the use of second-order nonlinear optical techniques. Since the second-order susceptibility Since the energies of the vibrational modes lie in the infra- vanishes in isotropic media (see Ref. [1] for a more detailed red region of the electromagnetic spectrum, infrared explanation), second-order nonlinear optical spectroscopy photons are needed to excite vibrational resonances. Also, can be used as an extremely sensitive surface probe. high electromagnetic field strengths are required to achieve A molecular picture of an interface can be obtained by enough response from the second-order susceptibility . using vibrational sum frequency generation (SFG). Here, (which is nonlinear and only occurs when pulsed lasers are ion an infrared (IR) and a visible (VIS) electromagnetic field, used). In recent decades femtosecond laser sources have ut with frequencies x2 and x1, are reflected from an interface. been built that can be used to generate infrared femtosecond ib At the interface a second-order polarization can be created laser pulses (with enough output energy for nonlinear opti- str di that oscillates at the sum frequency x0 of the incoming cal spectroscopy) with wavenumbers in the range 4000– waves: 1500 cm –1 or 2.5–7 lm. In this spectral range resonances onic of the localized surface chemical groups are probed, such tr ð2Þ ð2Þ as CH and OH groups, so that the structure, orientation Pi ðx0 ¼ x1 þ x2Þ¼vijk ðx0 ¼ x1 þ x2ÞE1;jðx1ÞE2;kðx2Þ 3 elec and order of small molecules can be mapped. Larger mole- r ð1Þ cules such as polymers, proteins and peptides however, are fo t still a challenge as they have complicated three dimensional o whose size and spectral shape is determined by the second- N structures that cannot be deduced from measuring only lo- order susceptibility tensor vð2Þ. If the energy of the IR beam s. calized subgroups of a larger structure. e is tuned around the resonance of the vibrational modes of t i Three dimensional structures can be deduced by probing the interfacial molecules the surface response is resonantly ts molecular vibrational modes that are comprised of the dis- enhanced. Since vð2Þ is a physical property of the material placement of a large number of atoms. These modes vibrate ane it must also reflect the spatial symmetry properties of that –1 tr with lower frequencies, from 1500–500 cm (7–20 lm). in material (Neumann’s principle). As a consequence (in the r ð2Þ In order to generate femtosecond infrared pulses with en- o electric-dipole approximation) vijk vanishes in bulk media t ough energy for nonlinear optical surface spectroscopy ex- [2]. This means that SFG can often be applied as a surface ne periments in the low frequency region of the infrared spec- r specific technique, with which only the first 1–3 molecular e t trum, we developed a modified Ti:Sapphire laser with a n layers situated at the interface are probed. The surface re- ð2Þ cryogenically cooled additional amplifier that pumps an op- ni sponse, identified as v , is usually split up in a frequency s tical parametric amplifier combined with difference fre- ei dependent resonant (res) and a frequency independent s quency generation stage (see Ref. [6] for details). Using non-resonant (nr) response: ru the pulses from this system we have measured the three di- fo t X mensional surface structure of a polysugar and the binding o ð2Þ ð2Þ ð2Þ iDu NshTiaTjbTkciRn;ab ln;c N vs;ijk ¼ vs;nr;ijk þ vs;res;ijk / Anre þ of a protein to a self assembled surface. n hðx2 À x0n þ i Ç0nÞ Figure 2 shows the surface localized (right panel) and de- ð2Þ localized (left panel) modes of two forms of a poly-(lactic de r. acid), here named form A and B. See Ref. [7] for more de- ijm where n refers to a specific vibrational mode, with reso- tails. This biodegradable and bio-compatible poly-sugar nance frequency x0n, and damping constant Ç0n. This has unique characteristics that heavily depend on the three ww. ð2Þ equation relates the resonant surface susceptibility vs;res dimensional structure of the molecule. It is an ideal candi- ð2Þ to the hyper-polarizability tensor bn which is the product date for use in systems and devices for drug delivery and of the vibrational dipole moment l with the Raman po- implants. Thorough knowledge of the three dimensional yw n larizability Rn through a transformation matrix T that surface structure is thus of great importance, since the sur- man transforms from the molecular coordinate frame (a, b, c) face constitutes a barrier to the surroundings and provides r e to the surface coordinate frame (i, j, k).
Details
-
File Typepdf
-
Upload Time-
-
Content LanguagesEnglish
-
Upload UserAnonymous/Not logged-in
-
File Pages7 Page
-
File Size-