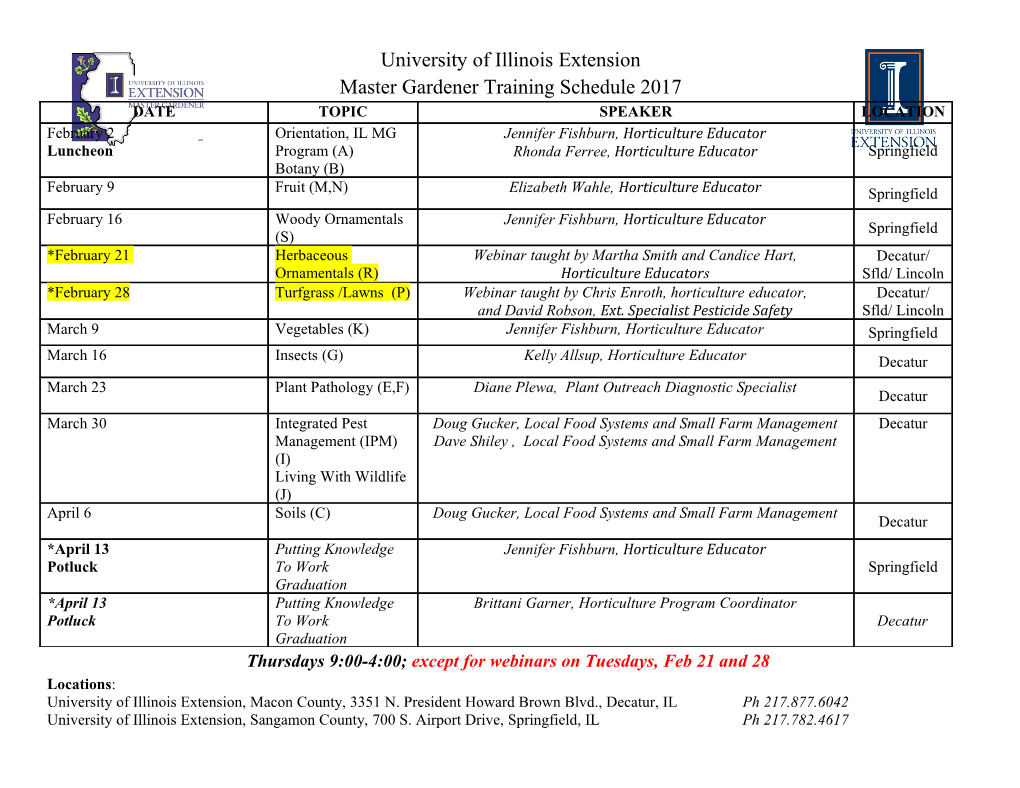
Organic Reaction Mechanisms Series SAMPLER INCLUDING Chapter 3: Oxidation and Reduction by R. N. Mehrotra from Organic Reaction Mechanisms 2015 Chapter 4: Carbenes and Nitrenes by E. Gras and S. Chassaing from Organic Reaction Mechanisms 2015 Discover more about the Organic Reaction Mechanisms Series: https://onlinelibrary.wiley.com/series/1200 CHAPTER 3 Oxidation and Reduction R. N. Mehrotra Formerly of Department of Chemistry, Jai Narain Vyas University, Jodhpur, India Reviews and Highlights ................................. 107 Oxidation by Metal Ions and Related Species ..................... 116 Magnesium .................................... 116 Chromium, Manganese, and Nickel ........................ 116 Copper, Silver, Gold, and Thallium ........................ 122 Cerium, Samarium, Titanium, Cobalt, Vanadium, Tungsten, Rhenium, and Molybdenum ................................. 129 Platinum and Iridium ............................... 135 Group VIII Metals: Iron, Palladium, Rhodium, Ruthenium, and Osmium .... 140 Oxidation by Compounds of Non-metallic Elements ................. 170 Sulfur and Boron ................................. 170 Halogens ...................................... 174 Peracids .......................................... 178 Ozonolysis and Ozonation ................................ 178 Singlet Oxygen ...................................... 183 Triplet Oxygen and Autoxidation ............................ 183 Other Oxidations ..................................... 184 Reductions ........................................ 189 Other Reactions ..................................... 193 References ........................................ 208 Reviews and Highlights A number of review articles, book chapters, and research reports were published during the year under review. A large number of these are related to aspects of oxidation followed by reduction including hydrogenation and transfer hydrogenation, and one each on hydroboration of carbonyl derivatives and hydrosulfurization and hydrodenitrogenation. 1 The review on the bio-inspired oxidations of aliphatic C–H groups with H2O2 in IV IV the presence of manganese complexes traces the first use ofL [ Mn (O)3Mn L](PF6)2 (L = 1,4,7-trimethyl-1,4,7-triaza cyclononane (Me3tacn)), the Wieghardt’s dinuclear Mn(IV) complex2 as the catalyst for the oxygenation of alkanes (hexane, cyclohexane, cycloheptane) in acetonitrile.3 The catalyst had 1350 turnovers in the presence of acetic acid, which is the best catalytic additive among the carboxylic acids. The Me3tacn- catalysed oxidation of cis- and trans-1,2-dimethylcyclohexanes in the presence of Organic Reaction Mechanisms 2015, First Edition. Edited by A. C. Knipe. © 2019 John Wiley & Sons Ltd. Published 2019 by John Wiley & Sons Ltd. 107 108 Organic Reaction Mechanisms 2015 sodium oxalate is highly stereo-retentive (>99% retention of configuration), which is considered as evidence against radical-type oxidation.4 The other Mn complexes that act as the catalyst in H2O2 oxidations are polymer- bound Mn(IV)-1,4,7-triazacyclonone for hydrocarbons,5 Mn complexes containing 1,4,7-trimethyl-1,4,7-triazacyclononane-derived ligands that are useful catalysts for the oxidation of olefins, alkanes, and alcohols,6 the Mn(II) complex with (1-(bis((pyridin- 2-yl)methyl)amino)-3-chloropropan-2-ol)(1-NO3)(2-NO3) which is a moderate catalyst in the oxidation of cyclohexane (upto 62 turnovers),7 the Mn(III) complex obtained by reacting the macrocyle ligand (1) with MnCl2, in the oxidation of various alkenes, alkanes, and aromatic compounds,8 and the complexes [((S,S)-N,N′-bis(2- ′ pyridylmethyl)-N,N -dimethyl-trans-1,2-diaminocyclohexane)Mn(CF3SO3)2] and (1S, ′ ′ 2S-N,N -dimethyl-N,N -bis((pyridine-2-yl)methyl)cyclohexane)Mn(CF3SO3)2)(A) in the oxidation of cis-1,2-dimethylcyclohexane.9 H O N C N N N N N C H O (1) The complexes [2-(((S)-2-((S)-1-((pyridin-2-yl)methyl)pyrrolidin-2-yl)pyrolidin-1- yl)methyl)pyridine Mn(CF3SO3)2], (A), and [N-methyl-N-((pyridin-2-yl)\methyl)((S)- 1-((pyridin-2-yl)methyl) pyrrolidin-2-yl)methanamine Mn(CF3SO3)2], (B), act as catalysts in the oxidation of a variety of secondary and tertiary alkanes in the presence of acetic acid (up to 970 catalytic turnovers). The cylcohexanol/cyclohexanone ratios of 4.9 : 5.1 point to a metal-based rather than radical-driven oxidation pathway.10a The complexes (A) and (B) also catalysed the enantioselective epoxidation of various olefins with AcOOH and H2O2/AcOH with high efficiency (up to 1000 turnover numbers (TONs)).10b The Mn complex with (N-methyl-N-((1-methyl-1H-benzo[d]imidazol-2- yl)methyl)((S)-1-((1-methyl-1H-benzo[d]imidazol-2-yl)methyl)pyrrolidin-2-yl)meth- anamine) is reported to catalyse the oxygenation of secondary benzylic and aliphatic C–H bonds in the presence of an acetic acid additive with the predominant formation of ketones (up to 154 TON).11 Further insights into the hydrogen-abstraction mechanism and oxygen-transfer step have been provided by a study involving the complex B and the complexes with the lig- ands 4-methoxy-2-((2-(1-((4-methoxy-3,5-dimethylpyridin-2-yl)methyl)pyrrolidin-2- yl)pyrrolidin-1-yl)methyl)-3,5-dimethylpyridine and 2-((2-(1-((4-(dimethylamino)-3,5- dimethylpyridin-2-yl)methyl)pyrrolidin-2-yl)pyrrolidin-1-yl)methyl)-N,N,3,5-tetra- methylpyridin-4-amine.12 The review on Pd(II)-catalysed oxidation of alkenes is focused mainly on (i) oxidations that proceed via cleavage of allylic C–H bond and the formation of a -allyl complex, 3 Oxidation and Reduction 109 (ii) Wacker-type oxidations that proceed via nucleopalladation followed by -hydride elimination, and (iii) 1,2-difunctionalization of alkenes that proceeds via nucleopalla- dation followed by functionalization of the resulting -alkylpalladium(II) intermediate. The mechanisms of each type of reactions are discussed with their scope and limitations for formation of new C–O or C–N bonds.13 The digest ‘Transition-metal-catalysed etherification of unactivated C–H bonds’ summarizes recent advances using different directing groups and catalyst systems for C(sp2)–H and C(sp3)–H bond activation. Despite the advances made, there are still some reactions that suffer from limited substrate scope, harsh reaction conditions, high catalyst loading, and the use of synthetically restricted directing groups. Some of the fundamental challenges such as (i) the development of an efficient catalyst system for the alkoxylation of unactivated C(sp3)–H bonds, (ii) the application of these transformations into the total synthesis of natural products, and (iii) an insightful understanding of the detailed mechanism that could help to design more efficient catalyst systems are yet to be addressed.14 Recent advances in the synthesis of chiral building blocks that use rhodium-catalysed asymmetric hydrogenation of olefins are discussed in a digest letter. Examples that have broadened the scope of the hydrogenation of enamides and enol esters are discussed in the first part, and the hydrogenation of substrates such as unsaturated carboxylic acids, nitroderivatives, or phosphonates, among others, are focused in the second part.15 A review titled ‘Quinone-catalysed selective oxidation of organic substrates’ focuses on the catalytic reactivity of quinones with high reduction potential, namely (2,3-dichloro- 5,6-dicyano-1,4-benzoquinone (DDQ)) and chloranil, which operate through a hydride abstraction mechanisms and typically react with electron-rich substrates capable of stabilizing carbocationic intermediates. The examples of single-electron transfer and addition–elimination reactions are also implicated with these quinones. Differences in the reactivity between DDQ and chloranil are typically rationalized on the basis of their differential reduction potentials, but the findings in the literature suggest that reactivities observed across diverse quinone structure types do not exhibit simple linear free-energy correlations. Instead, the reactivity is closely linked to the reaction pathways accessible to the different quinone structures. The second class of quinone consists of o-quinone catalysts that are shown to be com- patible with electrochemical and aerobic catalytic turnover. Mechanistic studies have shown that these quinones engage in electrophilic reaction pathways such as transam- ination and addition–elimination mechanisms, which introduce kinetically accessible pathways not readily available to the more oxidizing p-quinones. There has been signifi- cant progress in developing catalytic methods where the quinone is used in combination with a more desirable terminal oxidant.16 A brief summary of the recent developments in I2-catatalysed oxidative coupling reac- tions that utilize C–H and X–H (X = C, N, O, S, P, etc.) as nucleophiles and of the dif- ferent roles of the catalyst is presented in a review. The authors feel that enantioselective intermolecular reactions could be a promising development in future. The reaction con- ditions are generally mild and the compatibility of the substrate is good. Examples are considered with KI, n-Bu4NI (TBAI), I2, and different iodoarenes as the most used cat- alysts, while t-butyl hydroperoxide (TBHP), m-chloroperoxybenzoic acid (m-CPBA), H2O2, and AcOOH are the mostly used oxidants. Various reaction mechanisms are 110 Organic Reaction Mechanisms 2015 discussed in which different iodine species act as key intermediates. The mechanistic role of iodine catalysts is still largely unclear, though direct evidence of the reaction mechanism is available in a few examples.17
Details
-
File Typepdf
-
Upload Time-
-
Content LanguagesEnglish
-
Upload UserAnonymous/Not logged-in
-
File Pages143 Page
-
File Size-