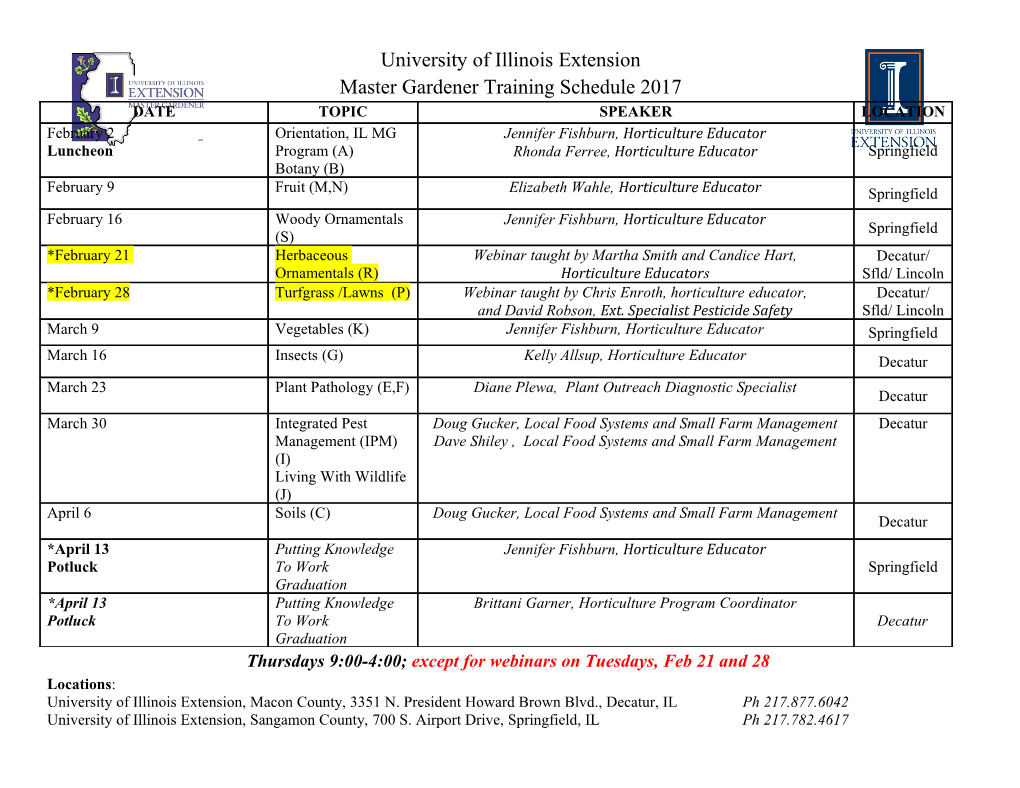
THE AMERICAN SOCIETY OF MECHANICAL ENGINEERS 35 E 7th St Yr Y 117 88^GT^^ E The Society shall not be responsible for statements or opinions advanced In papers or disci lion at meetings of the Society or of ias Division s or 1 Sections, or printed in its publications. Discussion is printed only if the paper is published in an ASME Journal. Authorization to photocopy for internal or personal use is granted to libraries and other users registered with the Copyright Clearance Center (CCC) provided $3/rtl r $/p pd t CCC d r nvr MA 193 t fr pl prn r bl rprdtn hld b ddrd t th ASME hnl blhn prtnt Copyright ® 1998 by ASME All ht rvd Printed in U.SA Downloaded from http://asmedigitalcollection.asme.org/GT/proceedings-pdf/GT1998/78644/V003T06A001/2411187/v003t06a001-98-gt-073.pdf by guest on 30 September 2021 EXPERIMENTAL AND NUMERICAL STUDIES OF NOx FORMATION IN TWO-STAGE METHANE-AIR FLAMES S.C. Li and F.A. Williams Center for Energy and Combustion Research Department of Applied Mechanics and Engineering Sciences University of California, San Diego La Jolla, CA 92093-0411 Presented at the International Gas Turbine & Aeroengine Congress & Exhibition Sthl Sdn — n -n 5 199 Downloaded from http://asmedigitalcollection.asme.org/GT/proceedings-pdf/GT1998/78644/V003T06A001/2411187/v003t06a001-98-gt-073.pdf by guest on 30 September 2021 ABSTRACT to the air stream. Results of the numerical inte- grations were in reasonable agreement with these To help understand how staged combustion aids experimental results when suitable selections were in reducing emissions of oxide of nitrogen from made of certain critical elementary reaction-rate gas turbines, measurements and computations constants. These new NO. measurements and are made of structures of two-stage counterflow computations help to increase understanding of methane-air flames at nomal atmospheric pres- influences of staging and diluent addition, iden- sure and a feed-stream temperature of about 300 tify important reactions for pollutant formation K. The fuel stream is partially premixed, with and suggest means to reduce emissions. equivalence ratios from 1.5 to 3.0. To the air stream is added up to 10% by mass of water spray, IOUCIO carbon dioxide, or nitrogen. Flame structures, in- cluding formation of species containing two car- Staged combustion is a well-established, practi- bon atoms, are measured by gas chromatography cal method for reducing emissions of oxides of of samples withdrawn by fine quartz probes and nitrogen (NO.) from gas turbine combustors [1- are calculated by numerical integrations of the 4]. Addition of inerts, such as H2O and CO2, conservation equations employing an updated el- for example through burnt-gas recirculation, also ementary chemical-kinetic data base. The same can be beneficial for this purpose. There remains, sampling system is employed with a low-flow-rate however, a lack of detailed understanding of the NOX analyzer to obtain profiles of nitric oxide and reasons that these techniques are so effective. Be- nitrogen dioxide, which are also calculated in the cause of this deficiency, it is difficult to optimize numerical integrations. The two-stage flame ex- combustors for achieving best performance with hibits a green fuel-rich premixed flame and a blue minimum emissions. The present work is designed diffusion flame with the maximum NO. concen- to increase knowledge of the fundamental factors trations found near the blue flame. At an air-side that determine the effects of staging and diluent strain rate of 50 s -1 , for fuel-side equivalence ra- addition, thereby aiding rational approaches to tios of 1.5, 2.0, 2.5 and 3.0, respectively, measured improvements in design of combustors. peak NO. concentrations were about 70, 90, 100 The results reported here represent a continu- and 90 ppm, reduced to 60, 770, 50, and 40 ppm, ation of our earlier efforts [5] to probe two-stage respectively, when 5% water by mass was added combustion. As in that work, methane was se- V, lected for the fuel. There are two principal rea- of the sum of C2H2, C2H4 and C2H6, the three sons for studying methane. First, it is the sim- major "C2 species"formed in methane flames, for plest model hydrocarbon fuel and thereby serves an air-side strain rate of a = 100 s-1 in the coun- as a starting point for investigating other hydro- terflow flames. The C2 species are important carbons [6, 7]. The chemical kinetics of methane both as soot precursors and as the main source combustion are less uncertain than those of other of the CH2 and CH radicals that are responsible hydrocarbons, so that key elementary steps and for the "prompt" mechanism of NO production. rate constants can be determined more accurately, The numerical computations with detailed chem- Downloaded from http://asmedigitalcollection.asme.org/GT/proceedings-pdf/GT1998/78644/V003T06A001/2411187/v003t06a001-98-gt-073.pdf by guest on 30 September 2021 and the fuel chemistry of higher hydrocarbons istry were found to overpredict the concentrations can be treated in a manner analogous to that of of C2 species by about a factor of two. Although methane, so that the results for methane indicate computational results also were reported for NO what needs to be studied for those other fuels. concentration profiles, it was concluded that be- Second, methane is the principal component of cause of existing uncertainties and the disagree- natural gas, which is becoming of increasing im- ments in profiles of C2 species, the reaction mech- portance as a gas-turbine fuel [8]. Appreciable anism needed revision in the future. No measure- practical interest thus is associated with methane ments of NO concentration profiles were made in combustion. this earlier work. The counterflow configuration investigated here The present paper reports an improved is illustrated schematically in Fig.1. The flames chemical-kinetic elementary data base as well are steady and planar, facilitating probe measure- as experiments for an air-side strain rate of a ments. The premixed flame at the top is observed =50 s -1 . Measurements are made of profiles of to be green, largely as a consequence of C2 band temperature, of concentrations of major stable emissions, while the diffusion flame is blue be- species, of concentrations of the sum of C2H2 and cause of nonequilibrium radiation emitted by ex- C2H4, and of the concentration of C2H6, as well as cited CO2 formed in CO oxidation. The experi- of NO and total NO R , over a range of equivalence mental arrangement has been described in detail ratios of the rich premixed flame, both with and previously [5] and therefore will not be discussed without water addition to the air stream. Com- thoroughly here. Related earlier investigations putational results with the improved chemical- in counterflow configurations also have been re- kinetic data are shown to be in reasonable agree- viewed previously [5]; hence that background in- ment with measurements, including those for C2 formation need not be repeated. It is, however, species and for NO.. Reliable descriptions of the worth stating that NO. measurements also have flame structures and NO production thereby are been reported for laminar coflow flames, both obtained. The results are employed to calculate methane diffusion flames [9] and methane [10] and the influences of the equivalence ratio and inert ethane [11] partially premixed flames; while these addition on the emission index for these two-stage experiments have aspects in common with those flames. addressed here, their nonplanar geometry causes the measurements and the interpretations of ex- EEIME perimental results to be more complicated for the coflow flames. The counterflow burner, which has two coax- The previous work [5] involved measurements of ial ducts placed one above the other, is concentration profiles of main stable species and shown schematically in Fig.1. The single-phase 3 methane-air gas mixture flows through the upper measurement techniques were discussed in previ- duct and the water spray with air through the ous publications [5, 12]. lower duct. The separation distance between the A O analyzer (model 955, Rosemount An- upper and lower duct exits is 18 mm. The up- alytical Inc.) was employed for measuring con- per duct has an exit diameter of 45 mm, and flow centrations of O and total O (the sum of the straighteners produce a uniform gas flow at the concentrations of NO and O The analyzer exit plane. The lower duct is a long contoured can be operated at a low sample flow rate so that tube with the same exit diameter. An atomizer the disturbance to flame structure can be reduced Downloaded from http://asmedigitalcollection.asme.org/GT/proceedings-pdf/GT1998/78644/V003T06A001/2411187/v003t06a001-98-gt-073.pdf by guest on 30 September 2021 is located at the bottom of the lower duct to pro- to an acceptably small level. The quartz micro- duce water spray. The air enters the duct below sampling probe for the analyzer is a standard thin, the spray nozzle and carries the spray upward to uncooled type as described by Saito et al. [13], and meet the gas flow from the upper duct. Since it is mounted on a two-dimensional positioner to some droplets are lost to the walls or to the bot- fix the probe at the desired location. The inner tom of the lower duct, a drain pipe was installed diameter of the probe tip is smaller than 0.1 mm. to discharge the accumulated liquid. The mass Samples were withdrawn from the flame by the flow rate of water in the counterflow was obtained analyzer continuously at a flow rate of approxi- as a function of the flow rate of the carrier gas by mately 0.4 cm3 /sec.
Details
-
File Typepdf
-
Upload Time-
-
Content LanguagesEnglish
-
Upload UserAnonymous/Not logged-in
-
File Pages23 Page
-
File Size-