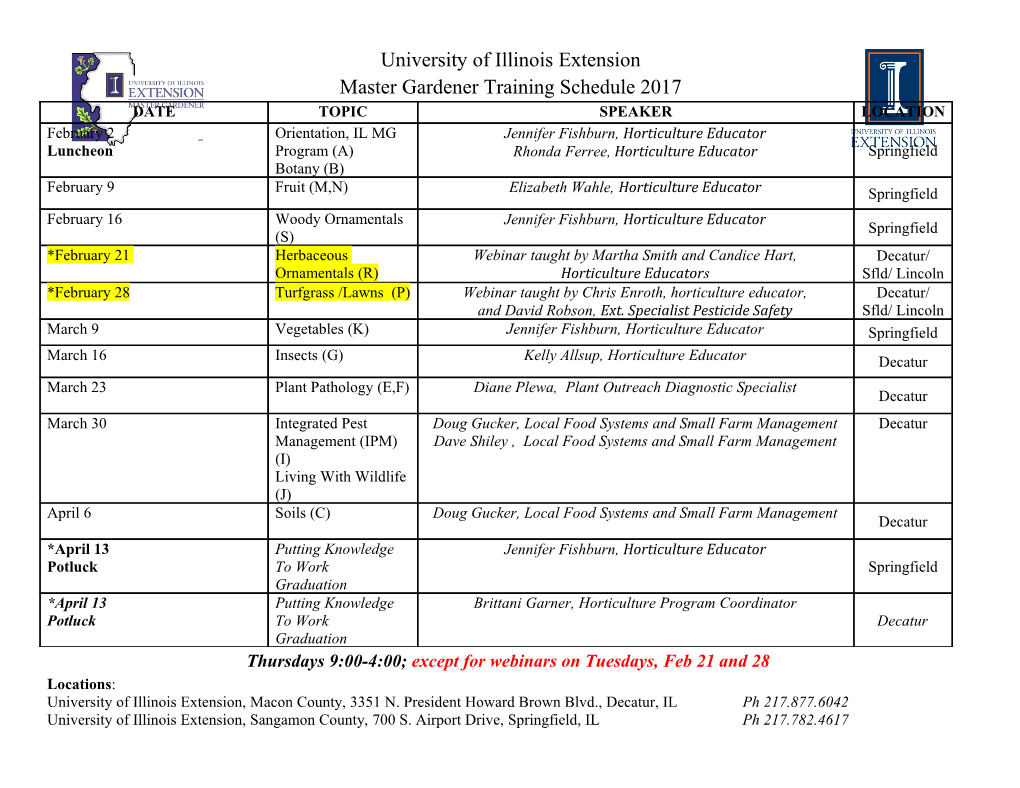
8 Behavioral Plasticity to Risk of Predation: Oviposition Site Selection by a Mosquito in Response to its Predators Leon Blaustein1, 2 and Douglas W. Whitman3 1Community Ecology Laboratory, Institute of Evolution, Department of Evolutionary and Environmental Biology, Faculty of Sciences, University of Haifa, Haifa 31905, Israel. 2Center for Vector Biology, Rutgers University, New Brunswick, New Jersey 08901, USA. E-mail: [email protected] 34120 Department of Biological Sciences, Illinois State University, Normal, IL 61790, USA. E-mail: [email protected] Abstract Oviposition habitat selection in response to risk of predation is an environmentally induced phenotypic plastic response. We suggest predator- prey characteristics for which such a response is more likely to evolve: high vulnerability of progeny to the predator; deposition of all eggs from a single reproductive event in a single site (i.e., inability to spread the risk spatially); few opportunities to reproduce (i.e., unable to spread the risk temporally); during habitat assessment by the gravid female, high predictability of future risk of predation for the period in which the progeny develop at the site; the predator is common but some sites are predator-free. We summarize work done on a particular system—the mosquito Culiseta longiareolata Macquart and its predators in pool habitats, a likely candidate system for oviposition habitat selection in response to risk of predation given these proposed characteristics. Adult C. longiareolata females can chemically detect various predatory backswimmer species (Notonectidae) but do not appear to chemically detect odonate and urodele larvae. Evidence suggests however that ovipositing females may detect other predators by nonchemical cues. C. longiareolata females can also detect and respond to larval food resource levels and conspecific larvae when choosing an oviposition site. When given only the two poor habitat choices of high conspecific larval densities or !& Phenotypic Plasticity of Insects Notonecta, overall oviposition rates drop and a larger proportion of the females oviposit in predator pools suggesting that females may operate in an ideal-free distribution manner. When comparing Notonecta versus non- predator pools, the proportion of egg rafts in predator-free pools increases when the proportion of predator-free pools increases. The most likely explanation among competing hypotheses is that the mosquito females that oviposit in predator pools are those that have encountered only predator pools when “sampling” the environment and ultimately choose to oviposit in a poor quality pool than no pool at all. A growing body of literature suggests that predator avoidance when ovipositing is particularly prevalent among pool/pond species with a mobile adult stage (amphibians and insects). We suggest that this is because risk of predation during the period in which offspring will grow is predictable based on risk of predation at the time that the female assesses habitats. Nonrandom selection of oviposition sites in response to predators likely influences adult populations and community structure. In the case of C. longiareolata, a model suggests that this behavior, compared to nonrandom oviposition, results in a larger adult population. Nonrandom selection of oviposition sites in response to risk of predation also indicates that experiments that set up predator and nonpredator plots and then compare the abundance of prey immatures are overestimating the predator’s effect on the prey population. Introduction Numerous studies over the past couple of decades have revealed a great diversity of induced responses by prey to risk of predation (see Whitman and Blaustein, this volume). The term “phenotypic plasticity” generally brings to mind developmental plasticity—e.g., altered growth rates (Peacor and Werner 2004), morphology (Trembath and Anholt 2001), life history (Blaustein 1997; Bernard 2004; Li 2002), or body color (Garcia et al. 2004). However, behavior, which is essentially an expressed phenotype of a genotype as a function of the environment, can also be considered a type of phenotypic plasticity (DeWitt and Scheiner 2004; Sih 2004). According to this definition, behaviors such as altered foraging (e.g., Kotler 1984; Brown and Kotler 2004) or altered oviposition site selection (Blaustein 1999; Resetarits 2005) in response to environmental conditions such as predation risk are examples of phenotypic plasticity. In general, behavioral plasticity allows a more rapid response and is generally less costly than induced morphological responses (e.g., Teplitsky et al. 2005). Moreover, behaviors are more easily reversible. Behavioral Plasticity to Risk of Predation !' That gravid females could detect risk of predation and choose a site for oviposition based on risk of predation was rarely examined in the literature several decades ago. This has been explored considerably more recently and there is a quickly growing body of literature that this behavioral plasticity is far from rare. A number of examples have been demonstrated for terrestrial insects (see Whitman and Blaustein, this volume). But this behavioral phenotypic plasticity is apparently common and particularly strong in pond and pool habitats (Blaustein 1999, Resetarits 2005). In this chapter, we first consider predator and prey characteristics in which evolution of this behavior is more likely to evolve. We then review a specific case of this behavioral phenotypic plasticity—oviposition habitat selection by a mosquito in response to risk of predation to its progeny. What Factors Favor the Evolution of Oviposition Plasticity in Prey? A species may evolve in two ways to avoid ovipositing where predators are prevalent. There may be evolutionary responses to predictors of predation risk without detecting the predator itself. For example, larger and more permanent pools are more likely to have higher predator densities (Spencer et al. 1999, Wilcox 2001, Chase and Knight 2003) and the ovipositing female may cue in on the size of the pool without being able to detect the predator itself. A second way is where detection of the predator itself induces a behavioral oviposition response. Not all species respond in this way to their predators. In fact, probably most do not (Blaustein et al. 2004). Certain predator-prey properties that likely increase the probability that oviposition habitat selection will evolve are outlined by Blaustein (1999) and Blaustein et al. (2004) and are expanded here: (1) A gravid female lays all her eggs in a single patch—i.e., she cannot spread the risk spatially. For example, the green toad, Bufo viridis, appears incapable of dividing its clutch and thus lays all its eggs in a single clutch while the tree frog Hyla savignyi may oviposit in several water bodies on the same night (Hill and Blaustein, unpublished data). Likewise, some mosquito species lay their entire clutch in a single egg boat whereas other mosquitoes may spread their eggs across several water bodies (Colton et al. 2003). A female that spreads her progeny across a number of sites is (by chance) likely to deposit eggs in both low- and high-quality habitats, and thus is likely to have at least some offspring survive. For the female that places all her eggs in a single site, site discrimination is more crucial to her fitness. ! Phenotypic Plasticity of Insects (2) A female has few lifetime reproductive events (cannot spread risk temporally). For example, some salamanders may live > 20 years, having at least one clutch per year (Warburg 1994). If a long-lived salamander deposits all of her progeny in a bad site during some years, it likely still has other opportunities. (3) High predator-induced mortality on immatures. In resource-limited habitats, moderate predation rates may even be beneficial by reducing intraspecific competition (e.g. Wilbur 1997), so vulnerability needs to be high for selective pressure favoring oviposition habitat selection. We would predict then that there would not be strong selection pressure for evolving oviposition habitat selection for prey species that have evolved successful ways of evading the predator when they co- occur at the same habitat. (4) The female has multiple sites from which to choose to oviposit and there is predator heterogeneity among patches. If suitable breeding patches are generally sufficiently distant, rare, or homogeneous, females may have evolved to oviposit into the first patch they encounter, without assessing patch quality. Otherwise, a female should search for a high quality site, though balancing costs of continued searching. (5) Predator abundance in a given site at time of oviposition predicts local predator abundance during subsequent larval development. In many systems, this is not the case. For example, an insect might oviposit on a leaf devoid of its predators or devoid of predator-released kairomones (Nakashima et al. 2004). But if the predator is mobile, then the likelihood of future risk of predation may not be sufficiently different on this leaf than a leaf that presently has a predator. On the other hand, many predators of discrete aquatic habitats or parasitoid larvae already in a host cannot move from patch to patch. In these cases, the present distribution of predators among sites at the time a female is searching for an oviposition site should be a good predictor of future predation risk for her offspring. (6) Immigration of prey individuals from geographic areas devoid of predators into areas having
Details
-
File Typepdf
-
Upload Time-
-
Content LanguagesEnglish
-
Upload UserAnonymous/Not logged-in
-
File Pages19 Page
-
File Size-