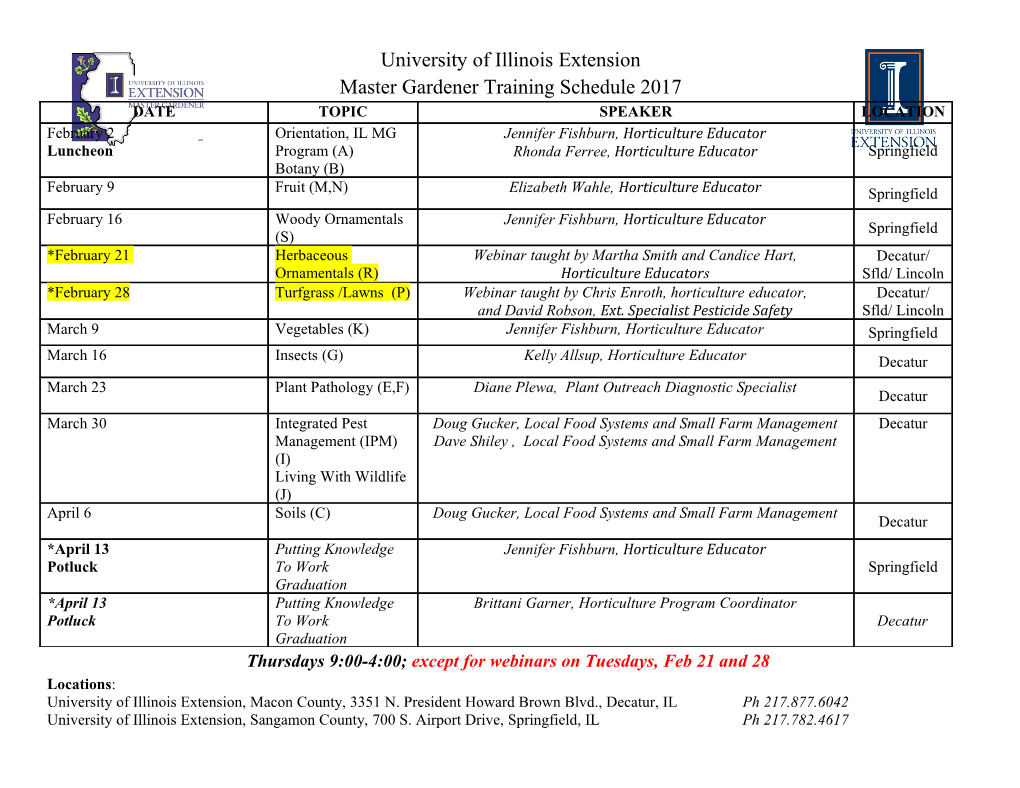
RESEARCH Nature of the Moho transition in NW Canada from combined near-vertical and wide-angle seismic- refl ection studies J. Oueity1,* and R.M. Clowes1 1DEPARTMENT OF EARTH AND OCEAN SCIENCES, UNIVERSITY OF BRITISH COLUMBIA, 6339 STORES ROAD, VANCOUVER, BC V6T 1Z4, CANADA ABSTRACT Although the year 2009 marked one century since the discovery of the Moho, or crust-mantle boundary, the exact nature of that boundary and the manner in which it formed remain major uncertainties in lithospheric studies. In northwestern Canada, sharp Moho refl ections at both near-vertical and wide-angle incidence have been imaged beneath the Great Bear magmatic arc. They show a remarkably fl at Moho and do not refl ect the complex tectonic history of the Wopmay orogen, of which the Great Bear arc is one part. In order to understand the origin of these refl ections and the nature of the Moho, we calculated near-vertical and wide-angle synthetic seismograms for a number of crust-mantle transition models using one- and two-dimensional wave propagation algorithms. Only laterally and vertically heterogeneous models can properly simulate the observed seismic signature recorded on both near-vertical and wide-angle refl ection data. The heteroge- neity is achieved by either laterally discontinuous layering or a lamellae structure with randomly distributed ellipses. These models suggest that the Moho represents a thermal/metamorphic front, a regional décollement, or both. LITHOSPHERE; v. 2; no. 5; p. 377–396. doi: 10.1130/L103.1 INTRODUCTION granulite, gabbro, and amphibolite and an upper mantle composed of sev- eral varieties of peridotite with some eclogite. The crust-mantle boundary, or the Moho, is one of the most distinct Despite all the geologic studies of the Moho by means of exposed sec- manifestations of a differentiated Earth. Major changes in petrology, min- tions (ophiolites) or xenolith samples, seismic data still remain the main eralogy, chemistry, seismic wave velocity, density, and rheology occur source for our understanding of the structure of the Moho and its develop- across it (Jarchow and Thompson, 1989). Originally, the Moho was defi ned ment. As techniques applied to lithospheric studies have improved con- on the basis of seismic head waves (Mohorovičić, 1910). It was described siderably in terms of seismic data acquisition technologies (e.g., Meiss- as the depth at which the compressional P-wave velocity increases rapidly ner, 1986; Mooney, 1987; Mjelde et al., 1997; Panea et al., 2005) and or discontinuously to 7.6–8.6 km/s (Steinhart, 1967). This suggested a pet- computational capabilities (e.g., Fuchs and Müller, 1971; Spence et al., rologic interpretation of the Moho as a fi rst-order (i.e., zero thickness) dis- 1984; Levander and Holliger, 1992; Zelt and Smith, 1992; Carbonell et al., continuity in rock composition from predominantly mafi c lower-crustal 2002), it has become apparent that the Moho is typically not a uniform, rocks to predominantly ultramafi c upper-mantle rocks. Ophiolite studies sharp, fi rst-order discontinuity, but rather a complex and variable transi- indicate that the lithologic sequence of the oceanic Moho includes pelagic tion zone. From the seismic perspective, a frequently cited model for the sediments, pillow basalts, massive and layered gabbro, and residual ultra- Moho is characterized by a variable-thickness transition zone composed mafi c tectonites of the upper mantle (Casey et al., 1981; Karson et al., of anastomosing layers progressing with depth from mafi c to ultramafi c 1984; Boudier and Nicolas, 1995; Jousselin and Nicolas, 2000; Dilek et rocks (Jarchow and Thompson, 1989; Braile and Chiang, 1986). Alterna- al., 2008). These rock cumulates form laterally discontinuous lenses with tively, the Moho transition may represent a change in scale of vertical lay- thicknesses ranging from 1 cm to several tens of meters and aspect ratios ering and horizontal extent of inhomogeneities (Tittgemeyer et al., 1999; ranging from 10:1 to 100:1. Prominent refl ections from within the oceanic Hurich, 2003; Carpentier and Roy-Chowdhury, 2007). Moho transition zone obtained by seismic-refl ection methods also support Although the Moho is well imaged by near-vertical incidence (NVI) a geologically complex boundary (e.g., Hasselgren and Clowes, 1995; and refraction/wide-angle refl ection (R/WAR) data, the exact nature and Nedimović et al., 2005). characteristics of the transition are not well understood. The wave fi eld Unlike abundant ophiolites, which allow direct observation of the oce- (i.e., waveform, duration, and amplitude) of a seismic signal (refl ected or anic Moho, exposed sections of the continental Moho are rare (Fountain refracted) from the lower crust and upper mantle may contain signifi cant and Salisbury, 1981). Nevertheless, important constraints on the nature information about the structure of the Moho transition zone, its fabric, and of the continental Moho are provided by the small continental data set scale of heterogeneities. Only a few studies have specifi cally addressed of xenoliths (McGetchin and Silver, 1972; Debari et al., 1987; Kopylova this issue. These studies can be grouped into two categories, qualitative et al., 1998). Xenolith samples indicate a lower crust composed of mafi c and quantitative. Examples of qualitative analyses of refl ection patterns near the Moho are those of Gibbs (1986) on Consortium for Continen- *Corresponding author: +1-604-822-5703; fax: +1-604-822-6088; e-mail: joueity@ tal Refl ection Profi ling (COCORP) seismic-refl ection profi les, Meissner eos.ubc.ca. (2000) using Deutsches Kontinentales Refl exionsseismisches Programm LITHOSPHEREFor permission to| Volumecopy, contact 2 | Number [email protected] 5 | www.gsapubs.org | © 2010 Geological Society of America 377 Downloaded from http://pubs.geoscienceworld.org/gsa/lithosphere/article-pdf/2/5/377/3050505/377.pdf by guest on 26 September 2021 OUEITY ET AL. (DEKORP) data, and Hammer and Clowes (1997), Cook (2002), and teristics of Moho refl ections by calculating the synthetic seismic signature Eaton (2006) on Lithoprobe refl ection transects that sample regions of for both near-vertical and wide-angle data of a number of crust-mantle diverse age and tectonic history. From these studies, refl ection patterns transition models using one- and two-dimensional wave propagation algo- appear to be divided into three primary classes: (1) a distinct, multicyclic rithms. The models range from a simple fi rst-order discontinuity to more band of refl ectors that are laterally continuous or piece-wise continuous complex, laterally and vertically heterogeneous structures. Unraveling over tens of kilometers, (2) fading out of crustal refl ectivity with depth, the detailed structure of the crust-mantle boundary and the way in which and (3) no clear refl ections in the vicinity of the Moho. These studies did it forms can help us understand some major tectonic processes, such as not involve wide-angle data. Although the comparisons have not clearly crustal growth, accretion, and delamination (Morozov et al., 2001; Car- linked a specifi c tectonic process with a distinct refl ection pattern, sharp bonell et al., 2002). Moho refl ections have been associated with lower-crustal deformational processes including compression, extension, and intrusive features. TECTONIC BACKGROUND Quantitative studies use synthetic modeling to construct the seismic response of the continental Moho using seismic velocity data (laboratory The Wopmay orogen in northwest Canada (Fig. 1) is a Paleoprotero- seismic-refraction results) together with petrologic information derived zoic assembly of domains that developed during the Calderian orogeny from exposures of the crust-mantle boundary when available. In earlier between 1.92 Ga and 1.84 Ga (Hoffman, 1989). The orogen is divided into studies, the Moho was modeled as a fi nite-thickness laminated zone of four major north-south–trending domains: the Coronation Supergroup, the alternating high- and low-velocity layers (Clowes and Kanasewich, 1970; Great Bear magmatic arc, the Hottah terrane, and the Fort Simpson ter- Hale and Thompson, 1982; Fountain, 1986; Braile and Chiang, 1986). rane. Rifting to the west of the Slave Province at ca. 1.90 Ga initiated More recently, the Moho has been considered as a laterally and vertically deposition of the Coronation Supergroup, a west-facing shelf rise and heterogeneous transition where the heterogeneities are represented by ran- foredeep succeeding prism, which shortly thereafter was intruded by a domly distributed ellipsoids (Carbonell et al., 2002; Tittgemeyer et al., suite of plutons and translated eastward by the Hottah collision to form a 2003). Another representation of the Moho follows a statistical approach, foreland fold-and-thrust belt (Hoffman, 1988). in which the transition zone is characterized by a stochastic heterogeneity The Hottah terrane formed as a magmatic arc and collided with the distribution (Hurich, 2003; Nielsen and Thybo, 2006; Carpentier and Roy- western Slave craton ca. 1.89–1.88 Ga (Hoffman and Bowring, 1984; Hil- Chowdhury, 2007). Only a few of these studies included consideration of debrand and Bowring, 1999). The collision caused compression, shorten- wide-angle data (Carbonell et al., 2002; Tittgemeyer et al., 2003; Nielsen ing, and eastward thrusting of the Coronation Supergroup onto the Slave and Thybo, 2006). craton (Hoffman and Bowring, 1984). The lack of coeval arc magmas on In this paper, we analyzed the near-vertical incidence and wide-angle the western Slave craton indicates that the accretion of the Hottah ter- seismic data acquired along line 1 of Lithoprobe’s Slave–Northern Cordil- rane onto the craton was the result of west-dipping subduction of an lera Lithospheric Evolution (SNORCLE) transect in the Paleoproterozoic– ocean basin. The Hottah terrane consists of Paleoproterozoic sedimentary Archean domains of the Northwest Territories, Canada (Cook et al., 1999; and intermediate volcanic rocks metamorphosed to amphibolite grade Fernández Viejo and Clowes, 2003). We investigated the dynamic charac- and intruded by calc-alkaline granitic plutons during the period 1.940– Cordillera Wopmay Slave 1100 CS N 64° Figure 1.
Details
-
File Typepdf
-
Upload Time-
-
Content LanguagesEnglish
-
Upload UserAnonymous/Not logged-in
-
File Pages20 Page
-
File Size-