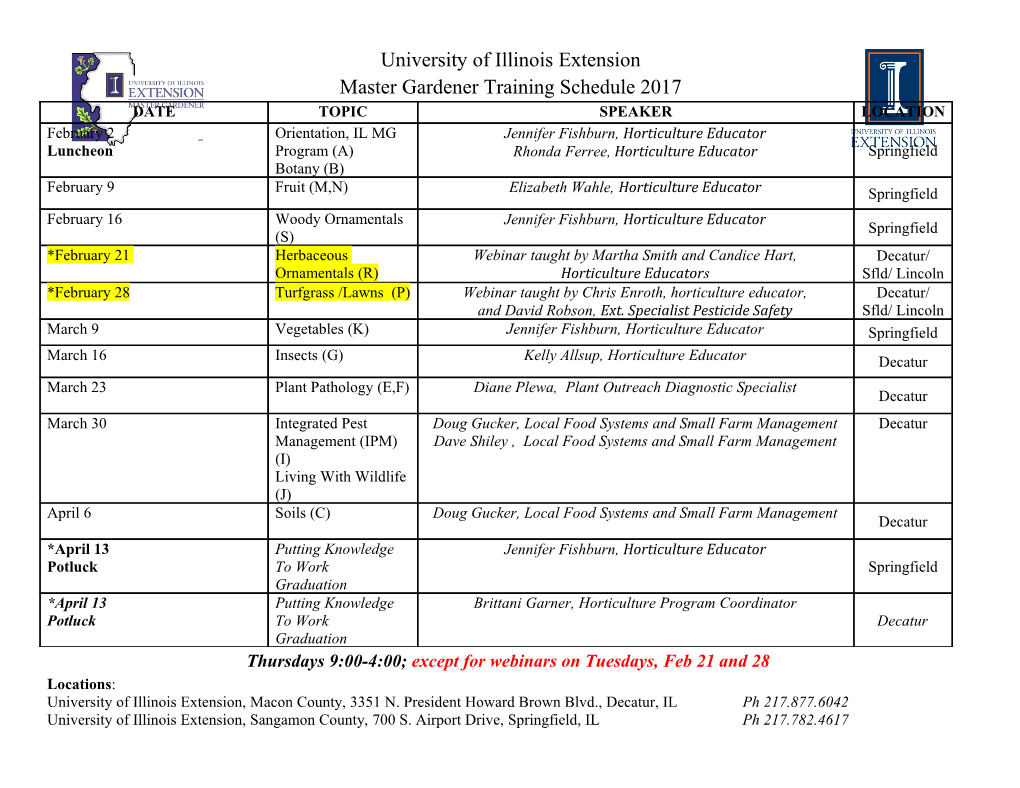
Appendix Functional Analysis and Operators A.1 Linear Space Definition A.1 A real linear space Z is a set {z1, z2,...} in which operations addi- tion ( + ) and scalar multiplication by real numbers are defined. The following axioms are satisfied for all zi ∈ Z and all scalars α, β ∈ R: 1 z1 + z2 ∈ Z 2 αz ∈ Z 3 z1 + z2 = z2 + z1 4 (z1 + z2) + z3 = z1 + (z2 + z3) 5. There exists 0 ∈ Z with the property that z + 0 = z for all z ∈ Z 6. For every z ∈ Z there is z˜ ∈ Z such that z +˜z = 0 7 (αβ)z = (βα)z 8 (α + β)z = (β + α)z 9 α(z1 + z2) = αz1 + αz2 10 1z = z The element 0 is known as the zero element. The scalars can also be the complex numbers, in which case it is a complex linear space. (Linear spaces are sometimes referred to as vector spaces.) The following can all be verified to be real linear spaces: n – R , vectors (z1, z2,...,zn) of length n with real-valued entries, – C[a, b], the set of real-valued continuous functions on an interval [a, b]; while the following spaces are complex linear spaces n – C , vectors (z1, z2,...,zn) of length n with complex-valued entries, © Springer Nature Switzerland AG 2020 253 K. A. Morris, Controller Design for Distributed Parameter Systems, Communications and Control Engineering, https://doi.org/10.1007/978-3-030-34949-3 254 Appendix: Functional Analysis and Operators – L2(a, b), the set of all scalar-valued functions f defined on (a, b) with b | f (x)|2dx < ∞ a and scalar multiplication by complex numbers. (This definition implies that f is well enough behaved that the above integral is well-defined.) A.2 Inner Products and Norms Consider solving a problem such as 1 k(s, t) f (s)ds = g(t) (A.1) 0 for the function f. The kernel of the integral, k, and the right-hand side g are known continuous functions. This has a passing similarity to a matrix equation Af = g, where matrix A ∈ Rn×n and vector g ∈ Rn are known while f ∈ Rn is to be calcu- lated. We know how to solve matrix equations, so trying to reformulate (A.1)asa matrix problem is tempting. √ The following is a basic result in Fourier series. The symbol j = −1 while α indicates the complex conjugate of α. Theorem A.2 For integers n = 0, ±1, ±2,...define j2πnt φn(t) = e = cos(2nπt) + j sin(2nπt). , = 1 φ ( )φ ( ) = 0 n m , 1. 0 n t m t dt , = 1 n m ∈ 2( , ) 1 ( )φ ( ) = 1 | ( )|2 = . 2. for any f L 0 1 , 0 f t n t dt 0 for all n implies 0 f t dt 0 This suggests writing ∞ f (t) = fi φi (t) (A.2) i=−∞ = 1 ( )φ ( ) . φ where fi 0 f t i t dt Multiplying each side of the above equation by m and integrating over [0, 1] yields, making use of property (1) above, 1 f (t)φm(t)dt = fm. (A.3) 0 Appendix: Functional Analysis and Operators 255 { }∞ Thus, for every continuous function f there corresponds an infinite vector fi i=−∞. Note that 1 1 ∞ ∞ 2 | f (t)| dt = fi φi (t) fm φm(t)dt 0 0 i=−∞ m=−∞ 1 ∞ = fi φi (t) fi φi (t)dt 0 i=−∞ ∞ 1 2 = | fi | φi (t)φi (t)dt i=−∞ 0 ∞ 2 = | fi | . i=−∞ The expansion (A.2) can be done for any function for which 1 | f (t)|2dt < ∞ . 0 In what sense is (A.2) valid? Define { fi } as in (A.3) and consider the error function N h(t) = f (t) − lim fi φi (t). N→∞ i=−N For any φm, 1 1 N h(t)φm(t)dt = fm − lim fi φi (t)φm(t)dt N→∞ 0 0 i=−N = fm − fm = 0. Property (2) in Theorem A.2 then implies statement (A.2) in the sense that the error 1 | ( )|2 = . h satisfies 0 h t dt 0 For notational convenience, renumber the indices: 0, −1, 1, −2, −2,...Defining f ={f0, f−1, f1, f−2, f2 ...}, ∞ 2 1 f =( | fi | ) 2 . (A.4) i=1 ∞ | |2 < ∞ . The linear space of vectors of infinite length for which i=1 fi is called 2 256 Appendix: Functional Analysis and Operators Returning to (A.1), assume g, f ∈ L2(0, 1). Replacing f, g by their Fourier series (A.2), 1 ∞ ∞ k(s, t) f j φ j (s)ds = g j φ j (t) 0 j=1 j=1 ∞ 1 ∞ k(s, t)φ j (s)dsfj = g j φ j (t). j=1 0 j=1 Multiply each side by φi (t) and integrate with respect to t over [0, 1], 1 ∞ 1 1 ∞ k(s, t)φ j (s)dsφi (t)dtfj = g j φ j (t)φi (t)dt 0 j=1 0 0 j=1 ∞ 1 1 k(s, t)φ j (s)dsφi (t)dt f j = gi . j=1 0 0 [A]ij Thus, defining ⎡ ⎤ ⎡ ⎤ g1 f1 ⎢ ⎥ ⎢ ⎥ 1 1 g = ⎣g2⎦ , f = ⎣ f2⎦ , [A] = k(s, t)φ (s)dsφ (t)dt, . ij j i . 0 0 ∞ [A]ij f j = gi j=1 or Af= g. An approximation to f can be calculated by solving the first N × N sub-block of the { }N infinite-matrix A for fi i=1, if this system of linear equations has a solution. Then, N fN (t) = fi φi (t) i=1 is an approximate solution to the integral equation (A.1). It needs to be shown that the error in approximating f by fN is small in some sense. This approach to solving an integral Eq. A.1 motivates the definition of an infinite “dot” product for infinite products for vectors in 2: Appendix: Functional Analysis and Operators 257 ∞ f · g = fi gi . (A.5) i=1 Also, ∞ f · g = fi gi i=1 ∞ 1 ∞ = fi φi (t) g j φ j (t)dt i=1 0 j=1 1 ∞ ∞ = fi φi (t) g j φ j (t)dt 0 i=1 j=1 1 = f (t)g(t)dt. 0 This suggests defining a “dot product” on L2(0, 1) by 1 f, g = f (t)g(t)dt. (A.6) 0 These scalar products are special cases of what is generally known as an inner product. Definition A.3 Let X be a complex linear space. A function ·, · : X × X → C is an inner product if, for all x, y, z ∈ X , α ∈ C, 1. x + y, z =x, z +y, z 2. αx, y =αx, y 3. x, y =y, x 4. x, x > 0ifx = 0 The definition for a real linear space is identical, except that the scalars are real numbers and property (3) reduces to x, y =y, x . An inner product space is a linear space together with an inner product. Examples of inner product spaces include 2 with the inner product (A.5), L2(0, 1) with the inner product (A.6). Some other inner product spaces are – continuously differentiable functions on [a, b] with the inner product 1 1 f, g = f (t)g(t)dt + f (t)g (t)dt 0 0 – continuous functions defined on some closed region ⊂ R3 with 258 Appendix: Functional Analysis and Operators f, g = f (x)g(x)dx. For vectors in Rn, the Euclidean inner product defines the length of the vector, n 2 1 x=( |xi | ) 2 . i=1 This concept extends to general inner product spaces. Definition A.4 A real-valued function ·:Z → R on a linear space Z is a norm if for all y, z ∈ Z, α ∈ R, 1. z≥0 (non-negative) 2. z=0 if and only z = 0 (strictly positive) 3. αz=|α|z(homogeneous) 4. y + z≤y+z (triangle inequality) For any inner product space, define 1 f =f, f 2 . (A.7) It is clear that the first three properties of a norm are satisfied. The last property, the triangle inequality, can be verified using the following result, known as the Cauchy– Schwarz Inequality. Theorem A.5 (Cauchy–Schwarz Inequality) For any x, y in an inner product space X, |x, y | ≤ xy where ·is defined in (A.7). Theorem A.6 Every inner product defines a norm. Although every inner product defines a norm, not every norm is derived from an inner product. For example, for a vector x in Rn, x∞ = max |xi | 1≤i≤n is a norm, but there is no corresponding inner product. The following result is now straightforward. Theorem A.7 (Pythogoreas) Let x, y be elements of any inner product space. x + y2 =x2 +y2 if and only if x, y =0. Appendix: Functional Analysis and Operators 259 Definition A.8 Elements f, g in an inner product space are orthogonal if f, g =0. Definition A.9 A linear space Z with a mapping ·:Z → R that satisfies the definition of a norm (A.4) is called a normed linear space. As noted above, every inner product defines a norm, so all inner product spaces are normed linear spaces. n The linear space R with the norm ·∞ is an example of a normed linear space. Other examples of normed linear spaces are – real-valued continuous functions defined on a closed bounded set ⊂ Rn with norm f =max | f (x)|, x∈ – L1(), the linear space of all functions integrable on ⊂ Rn, with norm f 1 = | f (x)|dx.
Details
-
File Typepdf
-
Upload Time-
-
Content LanguagesEnglish
-
Upload UserAnonymous/Not logged-in
-
File Pages35 Page
-
File Size-