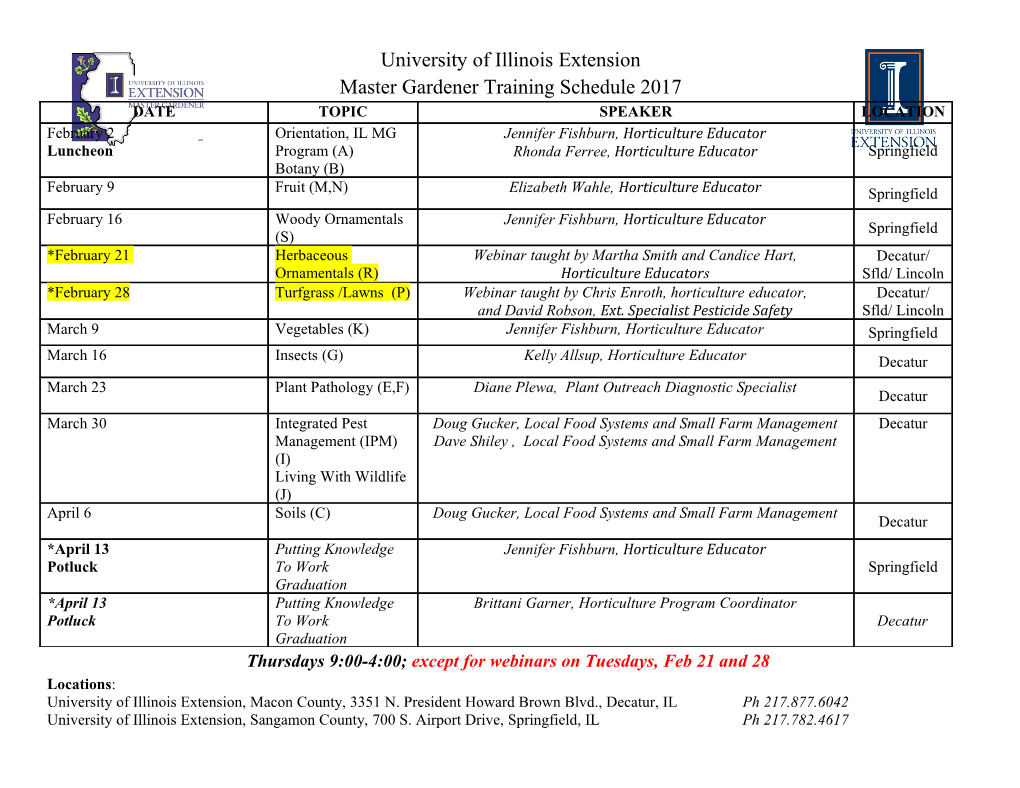
instruments Article Monte Carlo Modeling and Design of Photon Energy Attenuation Layers for >10× Quantum Yield Enhancement in Si-Based Hard X-ray Detectors Eldred Lee 1,2,*, Kaitlin M. Anagnost 1, Zhehui Wang 2 , Michael R. James 2, Eric R. Fossum 1 and Jifeng Liu 1,* 1 Thayer School of Engineering, Dartmouth College, Hanover, NH 03755, USA; [email protected] (K.M.A.); [email protected] (E.R.F.) 2 Los Alamos National Laboratory, Los Alamos, NM 87545, USA; [email protected] (Z.W.); [email protected] (M.R.J.) * Correspondence: [email protected] or [email protected] (E.L.); [email protected] (J.L.) Abstract: High-energy (>20 keV) X-ray photon detection at high quantum yield, high spatial reso- lution, and short response time has long been an important area of study in physics. Scintillation is a prevalent method but limited in various ways. Directly detecting high-energy X-ray photons has been a challenge to this day, mainly due to low photon-to-photoelectron conversion efficiencies. Commercially available state-of-the-art Si direct detection products such as the Si charge-coupled device (CCD) are inefficient for >10 keV photons. Here, we present Monte Carlo simulation results and analyses to introduce a highly effective yet simple high-energy X-ray detection concept with significantly enhanced photon-to-electron conversion efficiencies composed of two layers: a top high-Z photon energy attenuation layer (PAL) and a bottom Si detector. We use the principle of photon energy down conversion, where high-energy X-ray photon energies are attenuated down Citation: Lee, E.; Anagnost, K.M.; ≤ Wang, Z.; James, M.R.; Fossum, E.R.; to 10 keV via inelastic scattering suitable for efficient photoelectric absorption by Si. Our Monte Liu, J. Monte Carlo Modeling and Carlo simulation results demonstrate that a 10–30× increase in quantum yield can be achieved Design of Photon Energy Attenuation using PbTe PAL on Si, potentially advancing high-resolution, high-efficiency X-ray detection using Layers for >10× Quantum Yield PAL-enhanced Si CMOS image sensors. Enhancement in Si-Based Hard X-ray Detectors. Instruments 2021, 5, 17. Keywords: photonics; materials science; semiconductors; multidisciplinary; compound semiconduc- https://doi.org/10.3390/ tors; X-ray detection; image sensor; photon attenuation instruments5020017 Academic Editor: Antonio Ereditato 1. Introduction Received: 7 April 2021 Since the discovery of X-rays by Roentgen, the continuous expansion of X-ray tech- Accepted: 28 April 2021 Published: 30 April 2021 nology has transformed our society from materials science to biomedical applications. However, the capability gap for the efficient and direct detection of high-energy X-ray Publisher’s Note: MDPI stays neutral photons in the 20–50 keV range and beyond is challenging, which will prevent further with regard to jurisdictional claims in advancements in rising fields of technology such as the generic platform of X-ray imag- published maps and institutional affil- ing sensor technology and the next generation of synchrotron light source facilities [1–5]. iations. Scintillator-based methods are widely used in these types of facilities and high-energy X-ray detection technologies; however, they have major limitations such as decay time response and light yield [6,7]. Furthermore, the spatial resolution of such a method is limited due to the thickness required for the scintillator to absorb high-energy X-ray photons. There are also commercially available state-of-the-art X-ray detectors based on Si direct detection. Copyright: © 2021 by the authors. Licensee MDPI, Basel, Switzerland. For instance, the Si charge-coupled device (CCD) and CMOS image sensors, which are This article is an open access article commercially available in many formats, are known to be efficient in X-ray photon energies distributed under the terms and ≤10 keV and are typically most efficient in the 100 eV–10 keV range [8]. However, beyond conditions of the Creative Commons this energy, Si is notoriously inefficient in detecting X-rays as it is known to transmit the Attribution (CC BY) license (https:// majority of high-energy photons [8,9]. creativecommons.org/licenses/by/ There have been considerable efforts to overcome these issues and limitations by 4.0/). many researchers. One example of such work is a two-layer high-energy X-ray detec- Instruments 2021, 5, 17. https://doi.org/10.3390/instruments5020017 https://www.mdpi.com/journal/instruments Instruments 2021, 5, 17 2 of 17 tor structure comprising a cm-scale-area and mm-scale-thick metal buildup layer on a semiconductor thin-film to obtain high energy deposition and high dose delivery for radiation therapy [10,11]. However, because this type of work could be unsuitable with small-form-factor commercial-off-the-shelf technologies (COTs), such as CMOS image sensors (CIS) and quanta image sensors (QIS) as mm- and cm-scale metal thickness, could cause significant X-ray crosstalk to adjacent pixels that might be 20–50 µm in pixel pitch [12]. Furthermore, the claim that has been made for the underlying principle of this prior work is that high-energy X-ray photons are converted to secondary electrons through Compton interaction in the top mm-scale metal buildup layer followed by the transport of these sec- ondary electrons into the semiconductor thin-film layer for electron detection [10]. While this concept is intriguing, one questionable critical matter for our energy range of interest is that if the incident X-ray photon energies are relatively low compared to those intended for medical applications (MeV range), the low-energy secondary electrons (~1–10 keV range) could never escape the thick metal buildup layer after absorbing an X-ray photon as the corresponding electron mean free paths are rather small (<1 µm) in many materials [13]; therefore, the electrons could never make it to the bottom semiconductor thin-film layer for electron detection. Because of the relatively small travel ranges of electrons, even if the semiconductor thin-film layer for electron detection is decreased to µm-scale in terms of thickness, it is likely that the secondary electrons could still remain in the mm-scale-thick metal buildup layer. Similar to this example, a Si X-ray CT detector for MeV-range appli- cations that utilizes 200 µm-thick tungsten (W) metal sheet as a down-converter placed between two Si detectors has been previously developed and takes advantage of secondary electrons [14]. However, due to the thickness of the W layer, such a concept would be incompatible with and unideal for COTS CIS and QIS. Another class of work focuses on structured photocathode designs that depend on external photoemission, the angle of X-ray photon absorption, and the collection of elec- trons produced by photon interactions using an external electric field. This approach has demonstrated up to 5% quantum yield (QY), which is defined [15–17] as: # of primary photoelectrons collected QY = , (1) # of incident photons In this article, primary photoelectrons are defined as those directly excited by X-ray photons prior to impact ionization, while secondary photoelectrons are those created by primary photoelectrons via impact ionization [16]. For almost 4 decades, QY from external photoemission has been roaming around 1–1.5%; therefore, the increase to 5% is commendable [17,18]. While the technique to reach the 5% QY is claimed to be suitable for higher X-ray photon energies at around 20–30 keV, one should note that the X-ray photon absorption coefficient will decay rapidly with the increase in incident photon energies, which eventually will lead to lower QY at higher incident photon energies [8,9,19]. Therefore, this technique may not be extended to higher X-ray photon energy ranges of interest (i.e., >20 keV). There also have been numerous recent advances in the development of high-Z X- ray detectors such as Ge- and Cr compensated GaAs (GaAs:Cr)-based detectors [20–22]. However, these materials have considerable fabrication drawbacks. For instance, there is a necessity to eliminate thermally generated carriers by cooling the material down to cryogenic temperatures for Ge-based high-energy X-ray detectors [20,21]. Furthermore, while GaAs:Cr-based detectors seem favorable, fabrication yield of good quality GaAs can be difficult [22]. There also have been significant advances in CdTe-based detectors, which is now one of the most common materials used in high-energy X-ray detection. Some of the CdTe- based detectors report 75–100% efficiency at 20–50 keV range; however, to reach such high efficiency, the thicknesses of CdTe must be in hundreds of microns and commercial state-of- the-art CdTe-based detectors currently use mm-scale-thick CdTe layers [10,23,24]. It should also be stressed that unlike Si CIS-based devices, CdTe-based detectors cannot be easily Instruments 2021, 5, 17 3 of 17 scaled into large arrays. Moreover, large semiconductor material thicknesses will ultimately restrict the response time of the devices. As a result, relatively thick film applications will not be ideal and suitable for small-form-factor COTs CIS and QIS-based devices. In addition to the aforementioned research work and advances in high-energy X-ray detection methods, Si calorimeters have also been in extensively studied. While there have been significant efforts on the development of the Si calorimeters, including integration with CMOS-based sensors, they are typically for large-area Si applications (i.e., cm2 and m2) and also for considerably higher energy photons or particles, mostly in the GeV range [25]. Scintillators are known to have high absorption efficiencies. Similar to recent devel- opments in CdTe-based detectors, the absorption efficiencies may be between 50% and 100%.
Details
-
File Typepdf
-
Upload Time-
-
Content LanguagesEnglish
-
Upload UserAnonymous/Not logged-in
-
File Pages17 Page
-
File Size-