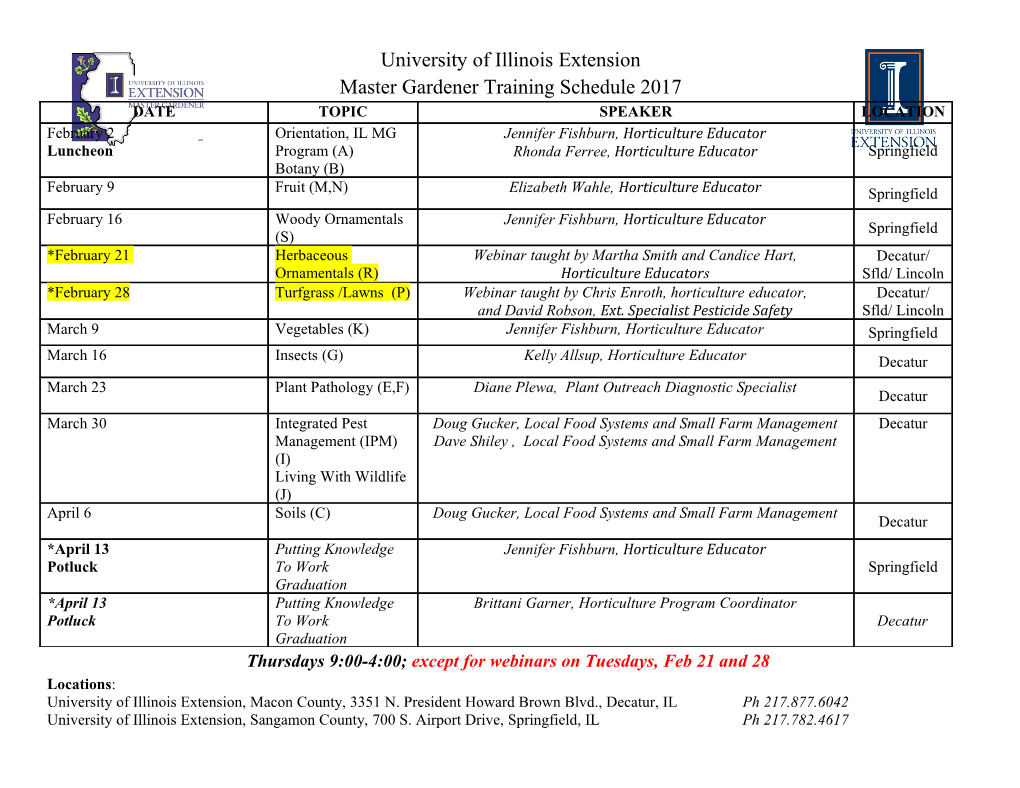
Theory and Application of SBS-based Group Velocity Manipulation in Optical Fiber by Yunhui Zhu Department of Physics Duke University Date: Approved: Daniel J. Gauthier, Advisor Henry Greenside Jungsang Kim Haiyan Gao David Skatrud Dissertation submitted in partial fulfillment of the requirements for the degree of Doctor of Philosophy in the Department of Physics in the Graduate School of Duke University 2013 ABSTRACT (Physics) Theory and Application of SBS-based Group Velocity Manipulation in Optical Fiber by Yunhui Zhu Department of Physics Duke University Date: Approved: Daniel J. Gauthier, Advisor Henry Greenside Jungsang Kim Haiyan Gao David Skatrud An abstract of a dissertation submitted in partial fulfillment of the requirements for the degree of Doctor of Philosophy in the Department of Physics in the Graduate School of Duke University 2013 Copyright c 2013 by Yunhui Zhu All rights reserved Abstract All-optical devices have attracted many research interests due to their ultimately low heat dissipation compared to conventional devices based on electric-optical conver- sion. With recent advances in nonlinear optics, it is now possible to design the optical properties of a medium via all-optical nonlinear effects in a table-top device or even on a chip. In this thesis, I realize all-optical control of the optical group velocity using the nonlinear process of stimulated Brillouin scattering (SBS) in optical fibers. The SBS- based techniques generally require very low pump power and offer a wide transparent window and a large tunable range. Moreover, my invention of the arbitrary SBS res- onance tailoring technique enables engineering of the optical properties to optimize desired function performance, which has made the SBS techniques particularly widely adapted for various applications. I demonstrate theoretically and experimentally how the all-optical control of group velocity is achieved using SBS in optical fibers. Particularly, I demonstrate that the fre- quency dependence of the wavevector experienced by the signal beam can be tailored using multi-line and broadband pump beams in the SBS process. Based on the theo- retical framework, I engineer the spectral profile to achieve two different application goals: a uniform low group velocity (slow light) within a broadband spectrum, and a group velocity with a linear dependence on the frequency detuning (group velocity dispersion or GVD). iv In the broadband SBS slow light experiment, I develop a novel noise current mod- ulation method that arbitrarily tailors the spectrum of a diode laser. Applying this method, I obtain a 5-GHz broadband SBS gain with optimized flat-topped profile, in comparison to the 40 MHz natural linewidth of the SBS resonance. Based on the ∼ broadband SBS resonance, I build a 5-GHz optical buffer and use this optical buffer to delay a return-to-zero data sequence of rate 2.5 GHz (pulse width 200 ps). The fast noise modulation method significantly stabilizes the SBS gain and improves the signal fidelity. I obtain a tunable delay up to one pulse-width with a peak signal-to-noise ratio of 7. I also find that SBS slow light performance can be improved by avoiding competing nonlinear effects. A gain-bandwidth product of 344 dB GHz is obtained in · our system with a highly-nonlinear optical fiber. Besides the slow light applications, I realize that group velocity dispersion is also optically controlled via the SBS process. In the very recent GVD experiment, I use a dual-line SBS resonance and obtain a tunable GVD parameter of 7.5 ns2/m, which is 109 times larger than the value found in a single-mode fiber. The large GVD system is used to disperse an optical pulse with a pulse width of 28 ns, which is beyond the capability for current dispersion techniques working in the picosecond and sub picosecond region. The SBS-based all-optical control of GVD is also widely tunable and can be applied to any wavelength within the transparent window of the optical fiber. I expect many future extensions following this work on the SBS-based all-optical GVD control using the readily developed SBS tailoring techniques. Finally, I extend the basic theory of backwards SBS to describe the forward SBS observed in a highly nonlinear fiber, where asymmetric forward SBS resonances are 1 observed at the gigahertz range. An especially large gain coefficient of 34.7 W− is ob- served at the resonance frequency of 933.8 MHz. This is due to good overlap between the optical wave and the high order guided radial acoustic wave. The interplay from the competing process known as the Kerr effect is also accounted for in the theory. v Acknowledgements The six years of my Ph.D. study in Duke Physics has been truly enjoyable in a quite unexpected way and this is of course due to the intelligent, passionate people I live and study with, and I can only name a few here. First and foremost, I want to thank my advisor, Prof. Daniel Gauthier, for his knowledge, mentorship and strict but patient guidance. I could never make it this far without his encouragement and incitations. I also want to thank Prof. Jungsang Kim and Prof. Mark Neifeld for their guidance on my research projects. I have enjoyed working with all of my colleagues in the Quantum Electronics Lab, and I want to thank all my collaborators, Edu Cabrera-Granado, Rui Zhang, Jing Wang, Myungjun Lee, Hannah Guilbert and Joel Greenberg. I have learnt a lot from cooper- ation in experiments and white board discussions. I also thank the non-optical half of the group who answered all of my electronic questions in the lab. Specific recognition goes to my thesis reader Hannah Guilbert, for insightful suggestions and tedious work on word editing. Finally, I thank my friends and family for making life easy and comfortable, par- ticularly my roommate Baiyu Chen, who helped me keep my life organized, and my wonderful husband Hang Yu who absorbed all my frustrations. I shall never forget what I learned and experienced during my years as a graduate student of physics. vi Contents Abstract iv Acknowledgements vi List of Figures xi List of Tables 1 1 Overview 1 1.1 Motivation: Slow light and design of the optical propertiesofmaterials. 1 1.2 Background...................................... 4 1.2.1 Stimulated Brillouin scattering in optical fiber . ........ 4 1.2.2 Groupindexandslowlight ....................... 6 1.2.3 Opticalbuffer................................ 8 1.2.4 Competingeffects ............................. 10 1.2.5 Groupvelocitydispersion . 12 1.2.6 ForwardSBS ................................ 13 1.3 Outline ........................................ 14 2 Theory of stimulated Brillouin scattering in optical fiber 18 2.1 Nonlinear opticalprocessesin an opticalfiber . ........ 19 2.1.1 Opticalfiber................................. 19 2.1.2 Nonlinearsusceptibility. 20 2.2 StimulatedBrillouinscattering . ....... 24 vii 2.2.1 Overallinterpretation . 24 2.2.2 Energy and phase matching conditions and Brillouin frequency ΩB 26 2.2.3 Acoustic-opticalcouplingin optical fiber . ...... 27 2.2.4 Single-linedSBSresonance . 29 2.2.5 Coupledintensityequations . 33 2.2.6 SBS with multiple-line and distributed broadband pump beam . 35 2.3 Physics interpretation of the SBS wavevector K ................ 37 2.3.1 Imaginary and real part of wavevector K ............... 37 2.3.2 Group velocity and group velocity dispersion . ..... 38 3 Broadband SBS slow light 41 3.1 IntroductiontobroadbandSBS. 42 3.2 TheoryofbroadbandSBSslowlight . 43 3.2.1 Slow light with a single-lined SBS gain resonance . ..... 43 3.2.2 Broadband slow light using a rectangular-shaped SBS resonance profile .................................... 46 3.2.3 LimitationduetoSBSgainsaturation . 51 3.3 Broadband optimal SBS gain profile design with direct current modulation 52 3.4 Slow-lightperformance ........................... 58 3.5 Conclusion ...................................... 60 4 Fast noise modulation method in high-fidelity broadband SBS slow light 61 4.1 Noisemodulationmethod........................... 63 4.2 Optimal SBS profile design using the fast noise modulationmethod . 65 4.3 Slow-lightperformance ........................... 73 4.4 Conclusion ...................................... 78 5 Competing effects in a broadband SBS slow light system 81 5.1 Competing processes in a broadband SBS slow light system ........ 84 viii 5.1.1 StimulatedBrillouinscattering . 84 5.1.2 Amplified spontaneous Brillouin scattering . ...... 86 5.1.3 Rayleighbackscattering. 88 5.1.4 StimulatedRamanscattering . 89 5.1.5 Modulationinstability. 90 5.2 Experimental study of competition between MI and SBS in a 10-GHz SBSslowlightsystem ............................... 91 5.3 ComparisonofMIwithSRS........................... 98 5.4 Conclusion ...................................... 100 6 SBS group velocity dispersion 101 6.1 Groupvelocitydispersioninmaterials . .......102 6.2 Theory of SBS-based group velocity dispersion . ........107 6.3 Experiment of SBS-based group velocity control . ........111 6.3.1 DispersiveSBSgainprofile. 111 6.3.2 Dispersionofa28-nsGaussianpulse. 113 6.4 Conclusion ...................................... 117 7 Forward SBS scattering in optical fiber 119 7.1 Introduction of forward stimulated Brillouin scattering...........120 7.1.1 Guidedacousticmodeinanopticalfiber . 121 7.1.2 CascadedForwardSBS .......................... 123 7.1.3 Acoustic-opticaloverlap . 125 7.2 Theoretical description of the FSBS and Kerr effect . .........127 7.2.1 Acoustic-optical coupling equation for the radial guided acoustic modes ....................................127
Details
-
File Typepdf
-
Upload Time-
-
Content LanguagesEnglish
-
Upload UserAnonymous/Not logged-in
-
File Pages191 Page
-
File Size-