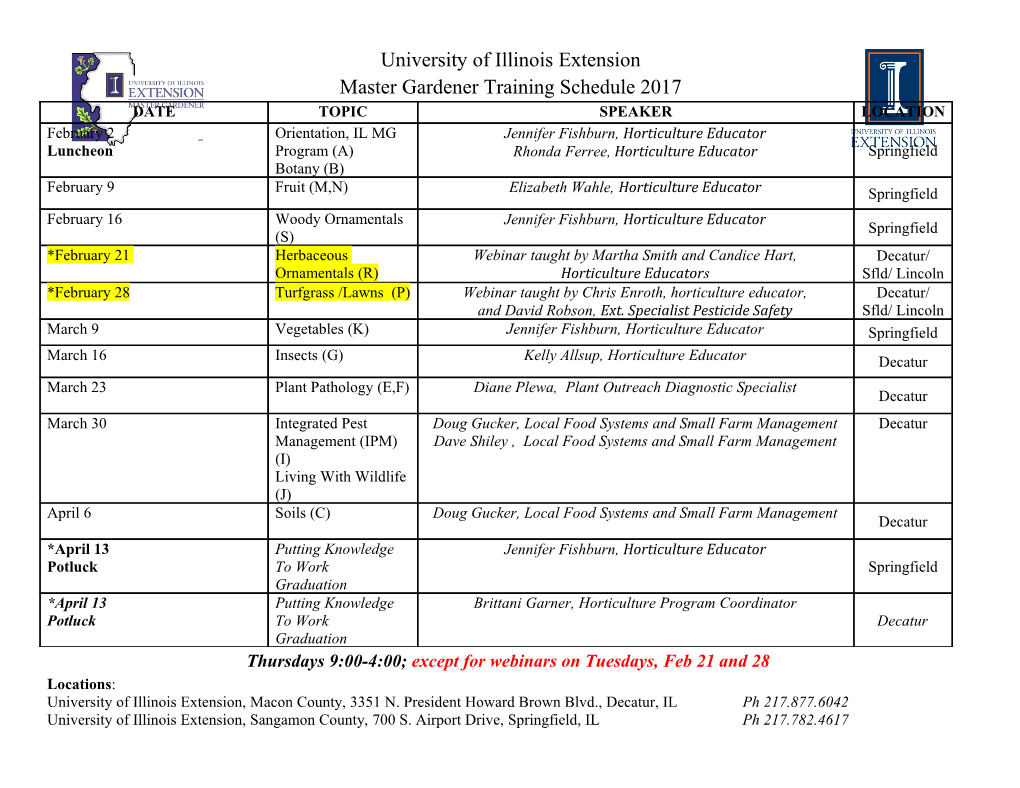
JCK(Wiley) RIGHT INTERACTIVE Detailed Kinetic Modeling of 1,3-Butadiene Oxidation at High Temperatures ALEXANDER LASKIN,1,* HAI WANG,1 CHUNG K. LAW2 1Department of Mechanical Engineering,University of Delaware, Newark, DE 19716-3140 2Department of Mechanical and Aerospace Engineering, Princeton University, Princeton, NJ 08544-5263 Received 31 October 1999; accepted 16 May 2000 ABSTRACT: The high-temperature kinetics of 1,3-butadiene oxidation was examined with de- tailed kinetic modeling. To facilitate model validation, flow reactor experiments were carried out for 1,3-butadiene pyrolysis and oxidation over the temperature range 1035–1185 K and at atmospheric pressure, extending similar experiments found in the literature to a wider range of equivalence ratio and temperature. The kinetic model was compiled on the basis of an extensive review of literature data and thermochemical considerations. The model was criti- cally validated against a range of experimental data. It is shown that the kinetic model com- piled in this study is capable of closely predicting a wide range of high-temperature oxidation and combustion responses. Based on this model, three separate pathways were identified for 1,3-butadiene oxidation, with the chemically activated reaction of Hи and 1,3-butadiene to produce ethylene and the vinyl radical being the most important channel over all experimental conditions. The remaining uncertainty in the butadiene chemistry is also discussed. ᭧ 2000 John Wiley & Sons, Inc. Int J Chem Kinet 32: 589–614, 2000 INTRODUCTION Brezinsky et al. [7] examined 1,3-butadiene oxi- dation in an atmospheric flow reactor at temperature The oxidation kinetics of 1,3-butadiene (1,3-C4H6)is of ϳ1100 K and equivalence ratios () of 0.55, 1.18, considerably important to the hierarchical develop- and 1.65. Their analysis revealed that the concentra- 9 " 9 ment of the kinetic mechanisms of hydrocarbon com- tion of crotonaldehyde (CH3 CH CH CHO) bustion. In several publications [1–3], we reported a peaked at an early reaction time. It was concluded comprehensive kinetic model of allene, propyne, pro- that crotonaldehyde was formed from 3-butenal " 9 9 pene, and propane combustion. This model uses GRI- (CH2 CH CH2 CHO) as a result of rapid isom- Mech 1.2 [4] as the C1-C2 kinetic subset, with the re- erization during passage through the GC injector sys- actions relevant to ethylene and acetylene combustion tem. Following this analysis, Brezinsky et al. [7] pro- carefully reexamined recently [5,6]. In the present posed that the oxidation of 1,3-butadiene starts mainly work, we report an experimental and kinetic modeling through O atom addition to the double bond to form study of 1,3-butadiene oxidation, a recent step in the 3-butenal. This is followed by its decomposition to direction towards a comprehensive and self-consistent allyl and CO: kinetic model of liquid hydrocarbon fuel combustion. ϩ !: 1,3-C46 H O " 9ии9 9 !: CH22CH CH CH O Correspondence to: H. Wang ([email protected]) " 9 9 !: CH22CH CH CHO *Current address: William R. Wiley Environmental Molecular " 9 9ииϩ Sciences Laboratory, Pacific Northwest National Lab., P.O. Box CH22CH CH CO H 999, MSIN K8-88, Richland, WA 99352. short ᭧ " 9 9ии!: ϩ 2000 John Wiley & Sons, Inc. CH22CH CH CO aCH 35CO standard long JCK(Wiley) LEFT INTERACTIVE 590 LASKIN ET AL It was also found that the H abstraction of 1,3-buta- relevant to 1,3-butadiene oxidation. These studies in- и и " diene by H and OH radicals to yield the CH2 clude rate-constant measurements for the reactions of CH9иC"CH (i-C H ) and CH "CH9CH" OOHؒؒand with 1,3-butadiene [32–34] and of и 2 4 5 2 CH (n-C4H5) isomers may also play a significant role ؒCH45radicals with O2 [35]. The thermochemical in butadiene oxidation. stability of the C4H5 radicals was also examined [36]. Reaction intermediates and products in two 1,3-bu- Finally, additional combustion data of 1,3-butadiene tadiene flames were determined by Cole et al. [8] using such as the ignition delay time [10] and the flame Molecular Beam Mass Spectrometry. These were lam- speed [37] were reported recently. inar, burner-stabilized flames, burning mixtures of 1,3- In this article, we present a detailed kinetic model butadiene, oxygen, and argon with equivalence ratios of 1,3-butadiene oxidation. The kinetic model was equal to 1.0 and 2.4. The emphasis of that work, as compiled by incorporating mechanistic and rate-con- well as the later kinetic modeling of these flames [9– stant information of C4HxOy species into the C3Hx 11], was the mechanism of aromatics formation. The mechanism published previously [1–3]. A large num- oxidation pathway, especially the mechanism of O- ber of the rate constants were estimated based on ther- atom addition to 1,3-butadiene, was left beyond the mochemical considerations and Rice-Ramsperger- focus of these studies. Kassel-Marcus (RRKM) [38,39] calculations using Recently, Dagaut and Cathonnet [12] published ex- potential energies and vibrational frequencies obtained perimental and modeling results of their study on the from molecular orbital calculations. For a critical val- oxidation of 1,3-butadiene in a jet-stirred reactor. Vi- idation of the model, we extended the flow reactor nyloxirane was observed in the postreaction mixtures. study of Brezinsky et al. [7] to a wider range of equiv- This finding prompted the authors to propose that the alence ratios and temperatures, using the same Prince- attack of the O atom on 1,3-butadiene leads primarily ton Turbulent Flow Reactor. The proposed model was to the formation of vinyloxirane, that is, validated against the experimental data of 1,3-butadi- ene oxidation in the flow reactor, shock tube, and ϩ и !: 1,3-C46 H O vinyloxirane flames. For all experimental conditions considered here, the The disappearance of vinyloxirane was assumed to oxidation of 1,3-butadiene was found to be controlled ϩ : ϩ proceed via unimolecular channels, mostly by Hؒ 1,3-C4H6 C2H4 ؒC2H3, followed -by the consumption of ؒC2H3 by O2. The O-atom at !: ϩ Vinyloxirane CH36 CO tack on 1,3-C4H6 also influences the overall oxidation !: ϩ CH24CH 2 CO process, but this influence is not as significant as the preceding reaction. The addition of the butadiene or through H abstraction by the radical pool. chemistry did not affect the predictive capability of The reaction pathway mentioned above is signifi- the C2-C3 model. cantly different from that of Brezinsky et al. [7], as the Dagaut-Cathonnet pathway leads to effective rad- ical-chain termination, whereas the pathway of Bre- EXPERIMENTAL DATA FOR MODEL zinsky et al. leads to effective chain branching. Despite VALIDATION this difference, both studies emphasized the dominant role of the reaction between 1,3-butadiene and the O Experimental data used for model verification are atom. For this reason, we conducted a critical review summarized in Table I. The data consist a total of 15 of the studies on the thermal reaction of various C4H6O cases, which range from pyrolysis and oxidation of isomers [13–20]. Our analyses led us to adopt and 1,3-butadiene in a flow reactor, to shock-tube pyrol- propose a viewpoint quite different from the previous ysis and ignition, and finally to laminar flames. The work. burner-stabilized flame of Cole et al. [8] was not in- A large volume of the fundamental kinetic data of cluded because the temperature profile of the flame is 1,3-butadiene oxidation has been accumulated over not known [9,11]. Below, we shall discuss the present the last decade. Significant progress was made in the flow-reactor experiments. Other experiments given in pyrolysis kinetics. Hidaka et al. [21] reviewed studies Table I are also briefly described. on the pyrolysis of 1,3-butadiene [22–27], 1,2-buta- diene [28,29], 1-butyne [30], and 2-butyne [31]. A ki- Flow Reactor Data netic model was proposed [21] which was capable of reconciling a wide spectrum of experimental data. In- The Princeton Turbulent Flow Reactor (PTFR), its short vestigations were also made on the elementary kinetics mode of operation, and the sampling system are de- standard long JCK(Wiley) RIGHT INTERACTIVE DETAILED KINETIC MODELING OF 1,3-BUTADIENE OXIDATION AT HIGH TEMPERATURES 591 Table I List of Experimental Data of 1,3-Butadiene High-Temperature Oxidation Used in Model Validation Reactant Composition, % Initial Conditions Case References/ a No. Experiment Type 1,3-C4H6 O2 Diluent T (K) p (atm) Comments 1a Flow reactor ϱ 0.3 — 99.7 1100 1 This work 1b 1150 1c 1185 2 Single-pulse shock tube ϱ 0.175 — 99.825(Ar) 1200–1800 6.5 Colket [27] 3 Single-pulse shock tube ϱ 0.5 — 99.5(Ar) 1200–1800 1.4–2.2 Hidaka et al. [21] 4 Flow reactor 1.63 0.142 0.48 99.378 1035 1 This work 5 Flow reactor 0.55 0.14 1.4 98.46 1035 1 This work 6 Flow reactor 4.7 0.14 0.12 99.74 1120 1 This work 7 Flow reactor 1.62 0.144 0.488 99.368 1110 1 This work 8 Flow reactor 1 0.14 0.78 99.368 1120 1 This work 9 Flow reactor 0.55 0.14 1.4 98.46 1120 1 This work 10 Flow reactor 1.65 0.143 0.477 99.38 1125 1 Brezinsky et al. [7] 11 Flow reactor 1.18 0.143 0.626 99.231 1125 1 Brezinsky et al. [7] 12 Shock-tube ignition 0.69 1 8 91(Ar) 1300–1500 8.5–10 Fournet et al.
Details
-
File Typepdf
-
Upload Time-
-
Content LanguagesEnglish
-
Upload UserAnonymous/Not logged-in
-
File Pages26 Page
-
File Size-