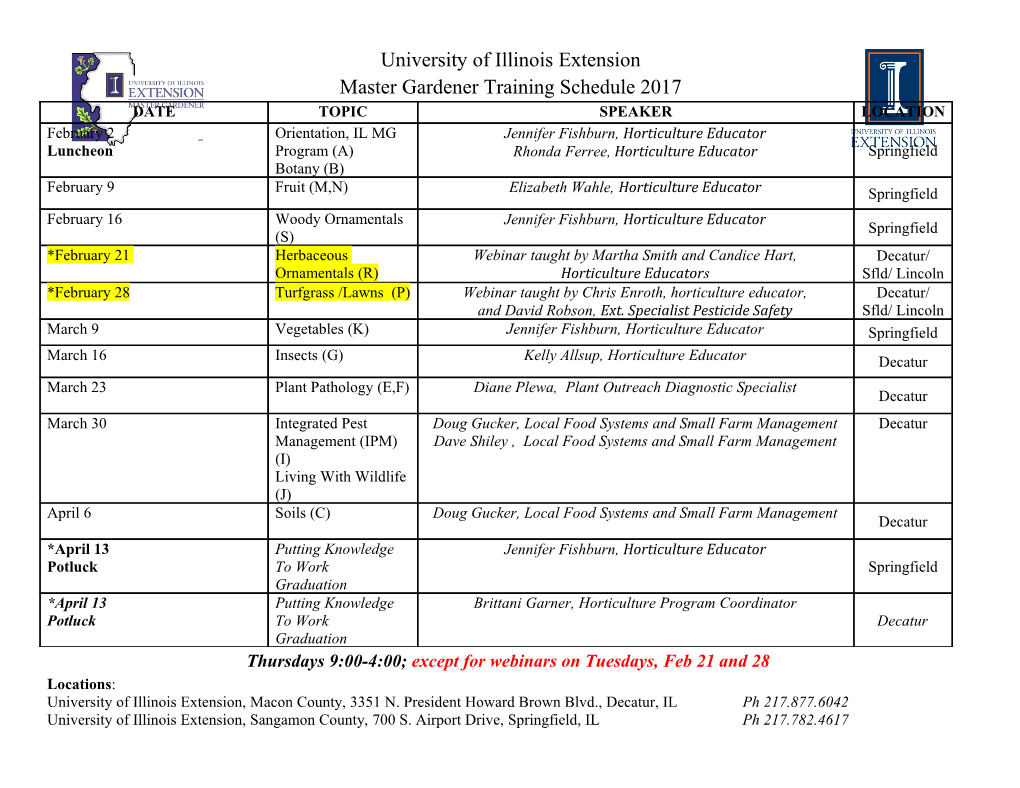
Advances in Space Research 35 (2005) 739–754 www.elsevier.com/locate/asr Solar radio emissions J.-P. Raulin *, A.A. Pacini 1 Centro de Radioastronomia e Astrofisica Mackenzie, Universidade Presbiteriana Mackenzie, Rua da Consolac¸a˜o 896, Sa˜o Paulo, 01302-907 SP, Brazil Received 3 June 2004; received in revised form 1 March 2005; accepted 1 March 2005 Abstract In this paper, we present a tutorial review which was presented at the first Advanced School on Space Environment (ASSE 2004). We first describe the basics of radioastronomy definitions, and discuss radiation processes relevant to solar radio emissions like plasma emission, free–free bremsstra¨hlung and gyromagnetic emissions. We illustrate these fundamentals by describing recent solar radio observations and the constraints they bring on different solar physical parameters. We focus on solar radio emissions from the quiet sun, active regions and during explosive events known as solar flares, and how the latter can bring quantitative informations on the particles responsible for the emission. Finally, particular attention is paid to new radio diagnostics obtained at very high fre- quencies in the millimeter/submillimeter range, as well as to radio emissions relevant to Space Weather studies. Ó 2005 COSPAR. Published by Elsevier Ltd. All rights reserved. Keywords: Solar radio astronomy; Radiation mechanisms; Solar flares; Submillimeter waves 1. Introduction During flares, energy stored in magnetic fields within ac- tive centers is rapidly converted into thermal, kinetic 1.1. Why do we study the Sun at radio wavelengths? and mechanical energies. As a consequence, the local plasma is heated to several or several tens of millions de- Although the Sun is an average (size, temperature grees, while other ambient particles are accelerated up to and brightness) middle-aged star, its proximity to Earth high energies. During large flares as much as few 1038 of about 150,000,000 km (1 AU) makes it an extraordi- electrons per second are accelerated, as deduced from nary laboratory for astrophysics. This proximity results non-thermal bremsstra¨hlung hard X-rays, which pro- in a very strong signal in almost all the electromagnetic vide a direct diagnostic of the electrons which collide spectrum, which allows to study the Sun with high sen- in the dense low solar atmosphere. Since a flare may last sitivity, time and spectral resolution. The SunÕs large few minutes, and assuming a typical coronal magnetic angular size of 320 as seen from the Earth also permits structure filled with 1037 thermal particles, we see that to describe in great details the solar surface and atmo- the accelerated electron production rate is very high. It spheric features. As a consequence, a wealth of phenom- is also difficult to know on the relative positions of the ena has been found to occur through the SunÕs acceleration regions (where the particles are accelerated) atmosphere. For example, the well-known solar flares and the radiation sites (where they emit). This raises the are sudden releases of a great amount of energy within question about the effects of the transport of these par- high magnetic field regions called solar active regions. ticles, on the observed time flare history, on the energy spectrum of the particles, if, for example acceleration and radiation sites are different. Solar flare radio obser- * Corresponding author. E-mail address: raulin@craam.mackenzie.br (J.-P. Raulin). vations give valuable informations in order to answer 1 Present address: Instituto Nacional de Pesquisas Espaciais, Sa˜o these questions. As it gives also access to the highest Jose´ dos Campos, Brazil. energy particles accelerated during flares, since high 0273-1177/$30 Ó 2005 COSPAR. Published by Elsevier Ltd. All rights reserved. doi:10.1016/j.asr.2005.03.138 740 J.-P. Raulin, A.A. Pacini / Advances in Space Research 35 (2005) 739–754 frequency radio diagnostics are more sensitive to these ative transfer of radio waves are discussed in Section 5, particles than are hard X-rays produced by non-thermal and in Section 6 we present selected solar radio observa- bremsstra¨hlung. Clues on the above mentioned ques- tions to illustrate the content of the previous sections. tions: particle number, energy and spectrum, transport Section 7 reviews and discuss some very high radio fre- of particles, are important to ultimately confront with quency solar observations, and in Section 8 we present the existing acceleration models (see Miller et al., 1997, radio observations relevant to Space Weather studies. for a review on models and how they are constrained In Section 9 we present our conclusions. by observations). During solar flares, large scale magnetic field struc- 1.2. How do we observe solar radio waves? tures can be destabilized, and be propelled into the interplanetary medium, along with the large masses it Radio astronomy developed during the last fifty contains, to form the so-called coronal mass ejections years, that is relatively early compared to X-ray, ultravi- (CMEs). It is now recognized that CMEs are the prin- olet and infrared techniques. The reason lies in the fact cipal drivers of the Space Weather and the near-Earth that most radio radiation penetrates down to the ground conditions. All these phenomena will emit at radio level. In Fig. 1, we show how the Earth atmosphere af- wavelengths in a frequency range covering over seven fects the incoming radiation from space as a function of orders of magnitude from few tens of kHz up to few its wavelength. We see that the atmosphere is completely tens or hundreds of GHz. Although many of the above transparent for waves between few centimeters and phenomena are well documented by the available 10 m. Below 0.5 mm the radiation is almost totally ab- observations, they raise questions that are still unre- sorbed. In the range 0.05–3 cm, there are some absorp- solved. For example, it is not clear at all what trigger tion regions mainly due to the presence of oxygen and the initiation of large CMEs. Plasma flows produced water vapour (Ulich, 1980), separated by windows of by filaments disruptions have been proposed (Wu variable transparency. At the other end of the radio et al., 2000), although they might not be a general spectrum, radiation with wavelengths greater than cause for all CMEs (Simnett, 2000). The relationship about 20 m are totally blocked by the EarthÕs between flares and CMEs is still a subject of discussion ionosphere. since many CMEs are detected without any flare man- To collect radio photons and concentrate them, ifestation. Although CMEs occurrence follows approx- radiotelescopes are used. These instruments can be imately the solar activity cycle, no clear associations single-dish antenna, as for example the 100 m diame- with the sunspot number have been found so far (How- ter Effelsberg radiotelescope shown in Fig. 2 and lo- ard et al., 1985; Webb, 2000). cated in Germany. The main limitation of such In the remaining part of this section, we briefly de- single-dish instruments are their poor capability of scribe how solar radio waves are observed. In the fol- resolving two distinct point sources on the sky, which lowing section, are presented basic definitions and is given by the angular separation a (in radians) relations useful in radio astronomy. Sections 3 and 4 are relative to radio emissions due to coherent and inco- k a ¼ 1.22 Á ; ð1Þ herent processes, respectively. Some aspects of the radi- D Fig. 1. Opacity of the Earth atmosphere as a function of wavelength (from NASA/IPAC). J.-P. Raulin, A.A. Pacini / Advances in Space Research 35 (2005) 739–754 741 techniques, the reader is referred to Kraus (1966), Chris- tiansen and Hogbom (1985), McLean and Labrum (1985). Since the solar spectrum varies in time, and since the spectrum of the radio emission can inform us about the emission mechanism, it is common to record the solar emission as a function of both frequency and time. Such way of representing the intensity of the radio emission is called a dynamic spectrum, shown for example in Fig. 4. Fig. 2. The 100 m diameter single-dish antenna radio telescope at Effelsberg (Germany). After aries.phys.yorku.ca/bartel/SNmovie/ Effelsberg.jp. 2. Radio astronomy: basic relations where k is the radio wavelength of observation and D is The radio emission received by a radio telescope is the diameter of the antenna. Thus, even observing the measured in terms of its intensity Im, which is the power Sun at a wavelength of 3 cm, the radiotelescope shown received per square meter, per frequency unit Hz, and in Fig. 2 will not be able to resolve solar features with per solid angle unit (Kraus, 1966). Integrating Im over 0 angular separation smaller than 1 , i.e., a projected size the solid angle subtended by the emitting source we on the solar surface of 42,000 km. Eq. (1) informs us get a quantity, Sm called the spectral flux density that higher spatial resolution can be obtained by using Z larger dishes, but clearly implying construction difficul- Sm ¼ Im dX. ð2Þ ties as well as high costs. To overcome this problem techniques have been developed based on the interfer- The unit of 1 W mÀ2 HzÀ1 is quite large for most of the ometry theory. The idea is to combine the signals from radio emitting astrophysical objects, and a new flux den- many radiotelescopes arranged as to form a linear array sity unit has been defined and called Jansky (Jy), with for example. The angular resolution of such an array is 1Jy=10À26 WmÀ2 HzÀ1. In solar radioastronomy, given by an equation similar as Eq. (1), where D is not and since the radio signal from the Sun is quite strong anymore the diameter of a single antenna, but the (few orders of magnitude stronger than the brightest greater distance between two individual antennae.
Details
-
File Typepdf
-
Upload Time-
-
Content LanguagesEnglish
-
Upload UserAnonymous/Not logged-in
-
File Pages16 Page
-
File Size-