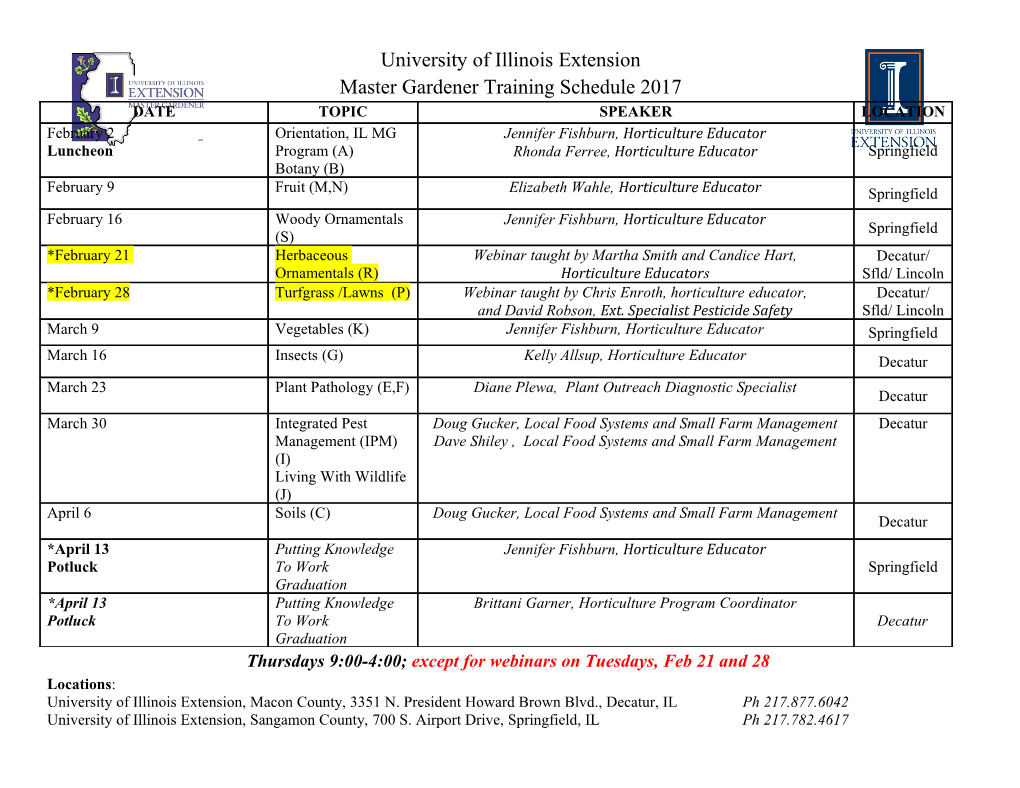
CdTe pixel detectors for the detection of the neutrinoless double beta decay for the COBRA experiment T. Gleixner, G. Anton, J. Durst, M. Filipenko, T. Michel ECAP Novel Detectors, Universit¨at Erlangen-N¨urnberg, Erwin-Rommel-Str. 1, 91058 Erlangen, Germany (Neutrinoless) double beta decay Influence of the detector parameters In the beta decay, one neutron decays into a proton, an electron and an electron antineutrino (A is To achieve a good sensitivity, a good energy resolution and detection efficiency is needed. There the number of nucleons, Z the number of protons): are several effects that contribute to the performance of the detector, and these are linked to the − (A,Z) → (A,Z +1)+ e + νe detector parameters. Parameters that can be influenced directly are the bias voltage, the thickness In the double beta decay, this happens with two neutrons: of the sensor, and the pixelpitch. With the current timepix, the pixelpitch can be varied in steps of − (A,Z) → (A,Z + 2) + 2e + 2νe 55 µm, by connecting only every n-th pixel electronic cell to the sensor. The main effects are charge The double beta decay is possible for several isotopes, for example 76Ge, 100Mo, 116Cd, 130Te, 136Xe. sharing (distribution of influenced currents on several pixel), recombination of charge carriers in the In the neutrinoless double beta decay (0nbb) no neutrinos are emitted: semiconductor, energy dependent noise of the (pixelwise) TOT counts (lower energy equals more (A,Z) → (A,Z + 2) + 2e− noise) and electrons leaving the sensor before depositing their whole energy. The main influence of This can be described as one electron antineutrino emitted by one of the neutrons, and absorbed as the detector parameter can be summarized as follows: an electron neutrino by the other neutron. This requires that the neutrino is a Majorana particle since • advantages of high bias voltage it must be identical with its antiparticle, and that it has a non-zero rest mass, since there must be a – less charge sharing because of lower drifttime change in helicity. If these requirements are fulfilled, this decay should be possible in the isotopes that – less trapping because of lower drifttime are capable of double beta decay. • disadvantages of high bias voltage The half-life of this decay is [1]: – limited by breakdown voltage 0ν 0ν 2 2 – leakage current increases 1/T1/2 = G |M | hmνi 0ν 0ν where G is the phase space integral, |M | the matrix element and hmνi the effective majorana • advantages of thicker sensors neutrino mass. So the detection of this decay would answer two fundamental questions: is the – less electrons leave the sensor neutrino a Majorana particle, and what is the effective Majorana neutrino mass? – the contribution of the energy carried by holes decreases, because of different weightingpoten- Since there are no neutrinos to carry away a part of the energy, the sum electron energy spectrum of tials this decay should be a peak around the Q-value. So most background can be eliminated by an energy • disadvantages of thicker sensors cut around the Q-value. – more charge sharing because of higher drifttime One difficulty with measuring this decay is the long half-life, which is probably above 1025 years. So – more recombination because of higher drifttime a detector to measure this decay requires a good energy resolution and must be able to surpress • advantages of a bigger pixelpitch background as efficiently as possible. – less charge sharing – higher energy per pixel, which decreases the noise of the TOT count • disadvantages of a bigger pixelpitch The Timepix detector – less information about the track, which makes the pattern recognition harder – noise increases (summing over less pixel) One concept of the COBRA experiment is to use pixelated CdTe detectors to measure the decay. The – the contribution of the charges carried by holes increases, because of different weightingpoten- double beta decay is possible in 116Cd which has a high Q-Value, and CdTe is a semiconductor that tials can be operated at room temperature. This allows a detector=source setup. Timepix [2] evolved from the Medipix2 [3], which was developed by the Medipix collaboration. It Because of these effects, there exists an optimum for the detector parameters. This can be determined consists of a pixelated sensor, each sensor pixel connected to an electronic pixel cell (55µm pitch). with Monte Carlo Simulations. Assuming a bias voltage of 500 V per mm sensor thickness (experiments CdTe enriched in 116Cd can be used as sensor material. A charged particle passing through the sensor showed that this is possible), one gets an optimum of about 3 mm sensor thickness and 165 µm pixel interacts with the sensormaterial, creating electron-hole pairs. To prevent these from recombining, an pitch, as shown below (track recognition is not included in these graphs, so the optimum pixelpitch is electrical potential difference between front- and back-side of the sensor is applied. This creates an lower then one might think from the graph). electrical field inside the sensor, along which charge carriers drift to the pixel electrodes, where they influence electrical currents. The pixel electronics shape them to voltage pulses. By measuring the time these pulses are 3.5 1.6e+26 0.0007 above a certain threshold (TOT=time 1.5e+26 3 over threshold measured in TOT counts), 0.0006 the energy deposited in each pixel by one 1.4e+26 2.5 0.0005 event can be determined. This means it 1.3e+26 is possible to measure the total deposited 0.0004 2 1.2e+26 energy of a particle by summing the en- 1.1e+26 1.5 ergy deposited in all triggered pixel and to 0.0003 events per kg keV a - in % energyresolution 1e+26 identify the particle (α, e , µ ...) by its 0.0002 1 9e+25 pattern of triggered pixel. On the right- limit for 3 sigma sensitivity as half-life 0.5 hand side, the simulated energy spectrum 0.0001 8e+25 of the (neutrinoless) double beta decay is 0 7e+25 0 100 150 200 250 300 350 400 450 500 550 2680 2700 2720 2740 2760 2780 2800 2820 100 150 200 250 300 350 400 450 500 550 shown, as it is seen by a timepix-like de- energy [keV] pixel pitch [mum] pixel pitch [mum] sensorthickness: 2 mm 3 mm 4 mm 5 mm sensorthickness: 2 mm 3 mm 4 mm 5 mm tector. neutrinoless decay decay with neutrinos Track recognition One of the main advatages of the small pixel pitch is, that it is possible to discriminate background by identifying the particles of an event. There are four main contributions to the background: Alpha particles, muons, single electrons, and two electrons from double beta decay with neutrinos. The signature of each of these is shown muon alpha double beta event single electron on the right. The muon and alpha signa- 120 255 400 220 ture was measured with a Timepix with a 118 silicon sensor and 55 µm pixel pitch, the 250 200 116 350 180 electron tracks were simulated for a pixel 245 114 300 160 pitch of 110 µm. While it is easy to iden- 112 240 140 tify alpha particles (round structure, high 250 110 235 120 energy deposition in the center) and muons 200 100 108 230 (straight line) by their characteristic signa- 150 80 106 tures, single electrons are not so easy. Dis- 225 100 60 104 criminating electrons from the double beta 40 220 50 decay with neutrinos, from those without 102 20 (by their tracks, assuming we already did a 100 0 215 0 190192194196198200202204206208210 160 165 170 175 180 185 190 cut for the energy), is impossible. The two tracks shown above look as would be expected (two/one ”head” with high energy where the path of the electrons ended, and a narrow track where the electron(s) were created). But not every track has to be so good, we only measure a two dimensional projection of their track, and the path of the electrons in the sensor is random. One concept to discriminate these two is pattern recognition with an artificial neural network. To do this, a set of n criteria is defined (for example the number of pixel with an energy above a threshold as one criteria), so from each event, a n-dimensional vector can be calculated. Currently there are ten criteria used. As an example the relative frequency of the number of pixel with an energy above the threshold is shown on the right. 0.09 1 Then the vectors of simulated events, where it is known if the track is from a single electron 0nbb or a double beta decay, can be used to train the network. The number -1 is assigned to 0.08 single 0.8 tracks from single electrons, +1 to tracks from double beta events. When the trained 0.07 network is given unknown vectors, it will calculate a number k between -1 and +1. If the 0.06 0.6 training was done correctly, a higher result will mean a higher chance for a track to be from 0.05 a double beta event. This can be seen in the figure on the far right. Shown on the x-axis is 0.04 0.4 50k + 50 (scaling is for technical reasons), on y-axis the relative frequency of values equal 0.03 frequency relative or smaller then 50k + 50. One of the lines is from the date used for training, the other is 0.02 relative frequency relative 0.2 independent. This is to make sure that there is no overtraining.
Details
-
File Typepdf
-
Upload Time-
-
Content LanguagesEnglish
-
Upload UserAnonymous/Not logged-in
-
File Pages1 Page
-
File Size-