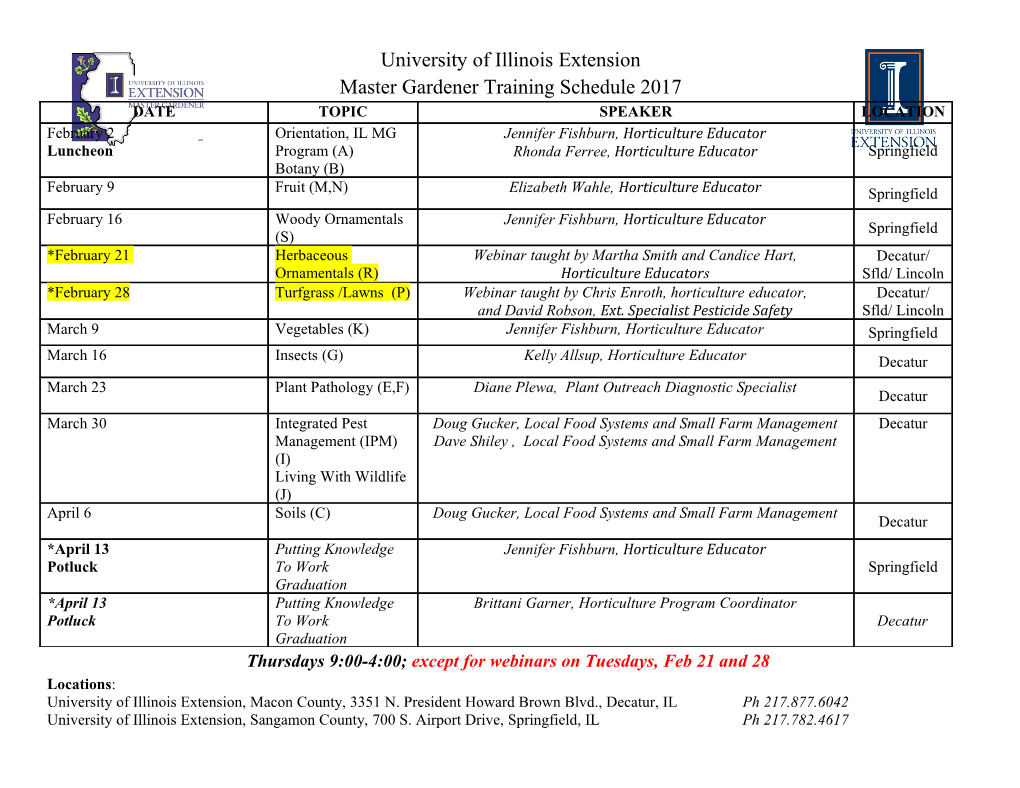
Structural basis for Ca2؉-independence and activation by homodimerization of tomato subtilase 3 Christian Ottmanna,1,2, Rolf Rosea,1, Franziska Huttenlocherb,1, Anna Cedzichb, Patrick Hauskea, Markus Kaisera, Robert Huberc,d, and Andreas Schallerb,2 aChemical Genomics Centre, Otto-Hahn-Strasse 15, 44227 Dortmund, Germany; bInstitute of Plant Physiology and Biotechnology, University of Hohenheim, 70593 Stuttgart, Germany; cMax Planck Institute for Biochemistry, 82152 Martinsried, Germany; and dZentrum fu¨r medizinische Biotechnologie, Universita¨t Duisburg-Essen, 45117 Essen, Germany Edited by Diter von Wettstein, Washington State University, Pullman, WA, and approved August 12, 2009 (received for review July 8, 2009) Subtilases are serine proteases found in Archae, Bacteria, yeasts, and 79 kDa glycoprotein that exhibits a remarkable level of stability higher eukaryotes. Plants possess many more of these subtilisin-like at elevated temperatures and alkaline pH (10). Like most other endopeptidases than animals, e.g., 56 identified genes in Arabidopsis subtilases, it is synthesized as a prepro-protein and targeted for compared with only 9 in humans, indicating important roles for secretion by an N-terminal signal peptide (10). Distinguishing subtilases in plant biology. We report the first structure of a plant features of SBT3 compared with mammalian PCs include the subtilase, SBT3 from tomato, in the active apo form and complexed C-terminal extension to the catalytic domain and, most notably, with a chloromethylketone (cmk) inhibitor. The domain architec- a large insertion between the His and Ser residues of the catalytic ture comprises an N-terminal protease domain displaying a 132 aa triad. This so-called protease-associated (PA) domain is also protease-associated (PA) domain insertion and a C-terminal seven- found in most other plant and some of the bacterial subtilases, stranded jelly-roll fibronectin (Fn) III-like domain. We present the first and in functionally unrelated proteins, including the mammalian structural evidence for an explicit function of PA domains in proteases transferrin and plant vacuolar-sorting receptors. Suggested func- revealing a vital role in the homo-dimerization of SBT3 and in enzyme tions for the PA domain include regulation of substrate access to activation. Although Ca2؉-binding sites are conserved and critical for the active site, ligand or substrate binding, and protein–protein stability in other subtilases, SBT3 was found to be Ca2؉-free and its interactions (11–14). The PA domain of SBT3 was found to be thermo stability is Ca2؉-independent. required for protease activity and secretion (10). As part of our efforts to clarify the function of the PA-domain and to identify calcium ͉ proprotein convertase ͉ protease-associated domain ͉ the factors governing SBT3 activity and stability, the enzyme was subtilisin ͉ thermostability crystallized and its structure elucidated. We report here the first structure of a subtilase from plants revealing a role for the PA ubtilases constitute the S8 family in clan SB of serine domain in homo-dimerization and regulation of protease activ- ity. Furthermore, we are unaware of another structure of a Sproteases (http://merops.sanger.ac.uk). They are character- ϩ 2 ized by a catalytic triad of Asp, His, and Ser residues in an subtilase that is Ca -free in its native state and independent of 2ϩ arrangement shared with subtilisins from Bacillus species (1). Ca with respect to activity and thermo stability. The first eukaryotic subtilase to be identified was kexin. It is Results and Discussion involved in the maturation of ␣-mating factor and killer toxin from their respective precursor proteins in yeast (2). Nine Overall Structure and Domain Architecture. SBT3 was purified from subtilisin-like endopeptidases have since been discovered in a tomato cell culture as the active enzyme (Thr-113 to Trp-761) mammals, seven of which are related to kexin and also involved with the signal peptide and the prodomain cleaved during in the highly specific processing of precursor proteins. Their zymogen maturation. We determined its structure by MIRAS substrates include polypeptide hormone precursors, growth fac- procedures and refined it at 2.5 Å (crystallographic statistics tors, receptors, enzymes, and viral surface glycoproteins, which given in Table S1) with details of the procedure described are typically cleaved on the carboxyl side of paired basic residues elsewhere (15). In the final model, density for 639 of 649 residues (3). The remaining two subtilases, PCSK9 and S1P, belong to the is well-defined. The polypeptide is folded into three domains, the proteinase K and pyrolysin subfamilies of subtilases (3). The catalytic (subtilisin) domain (Thr-113-Arg-342 and Leu-473-Tyr- discovery of mammalian proprotein convertases (PCs), charac- 654), an interposed protease-associated (PA) domain (Thr-343- terized by their exquisite substrate specificity compared with Tyr-472) and a C-terminal Fn III domain (Pro-655-Trp-761). bacterial subtilisins, further stimulated interest in this class of Concerning this domain architecture, the most similar protein is ␣ serine proteases. C5 peptidase from Streptococcus pyogenes [PDB entry 3EIF Plants appear to lack kexin-related PCs but they possess a (14)], which also displays a PA domain interposed in the protease largely expanded pyrolysin family with 56 genes identified in domain and shows three C-terminal Fn III-like domains the first Arabidopsis thaliana (4). They have been implicated in general protein turnover (5), the regulation of plant development (6), Author contributions: C.O. and A.S. designed research; C.O., R.R., F.H., A.C., and A.S. biotic and abiotic stress responses (7, 8), and the processing of performed research; P.H. and M.K. contributed new reagents/analytic tools; C.O., R.R., A.C., precursors of peptide growth factors in plants (9). It therefore R.H., and A.S. analyzed data; and C.O. and A.S. wrote the paper. seems that the majority of plant subtilases assumed plant-specific The authors declare no conflict of interest. functions in the course of evolution. With the physiological roles This article is a PNAS Direct Submission. of plant subtilases beginning to emerge, it will now be interesting Freely available online through the PNAS open access option. to see whether or not the adoption of specific roles in plant Data deposition: The atomic coordinates have been deposited in the Protein Data Bank, physiology is reflected in unique structural or biochemical www.pdb.org [PDB ID codes 3IS6 (apo SBT3) and 3I74 (cmk-inhibited enzyme)]. features that distinguish subtilases in plants from those in other 1C.O., R.R., and F.H. contributed equally to this work. organisms. 2To whom correspondence may be addressed. E-mail: [email protected] or To address this question we recently purified and character- [email protected]. ized a typical pyrolysin-like subtilase from tomato (Solanum This article contains supporting information online at www.pnas.org/cgi/content/full/ PLANT BIOLOGY lycopersicum) plants (SlSBT3 or SBT3). SBT3 is an extracellular 0907587106/DCSupplemental. www.pnas.org͞cgi͞doi͞10.1073͞pnas.0907587106 PNAS ͉ October 6, 2009 ͉ vol. 106 ͉ no. 40 ͉ 17223–17228 Downloaded by guest on September 25, 2021 Fig. 2. Superimpositions of individual SBT3 domains with their nearest structural homolog. (A) Ribbon plot of SBT3Јs subtilisin domain (red coil) superimposed with subtilisin BPNЈ (blue coil, PDB entry 1A2Q). Residues of the catalytic triad are shown as sticks colored in blue (BPNЈ) or for SBT3 in yellow (carbon), blue (nitrogen), and red (oxygen). Residue numbering of SBT3 is given as blue labels. Loops bordering the active site are labeled. A calcium ion bound by BPNЈ is shown as green sphere. (B) Ribbon plot of SBT3Јs PA domain (orange) superimposed with the apical domain of an aminopeptidase from Aneurinibacillus (cyan, PDB entry 2EK8). (C) Ribbon plot of the Fn III domain of SBT3 (blue) superimposed with SSP-19 (sperm-specific protein) from C. elegans (green, PDB entry 1ROW). is restricted by the PA-domain, enforcing a 90° kink in the Fig. 1. Overall structure of SBT3. (A) Stereoview of the ribbon plot of the putative substrate binding channel (Fig. 1B). SBT3 monomer. The catalytic (subtilisin), protease-associated (PA) and fi- Individual substrate binding pockets in the active site of SBT3 bronectin (Fn) III-like domains are colored red, orange and blue, respectively. were identified in the structure of peptidyl-chloromethylketone (B) Two perpendicular views of the solid surface representation of the SBT3 dimer with the subtilisin domains in red, the Fn III domains in blue, and the PA (Ac-Phe-Glu-Lys-Ala-cmk)-inhibited SBT3. The inhibitor is co- domains in orange and magenta. Active site residues are displayed as white valently bound to active site residues His-215 and Ser-538 (Fig. surface; N-linked oligosaccharides are displayed as gray sticks. The proposed S3A) and occupies the active site cleft in a way similar to other substrate binding channel is indicated by a dashed line. eukaryotic subtilases, for example kexin inhibited by Ac-Arg- Glu-Arg-Lys-cmk (16). The S1 pocket of the substrate-binding site is filled only partially by the P1-Ala side chain of the inhibitor of which is similar to the one in SBT3 (Fig. S1). SBT3 crystallizes and provides space for larger residues like asparagine or glu- as dimer with dimerization mediated by the PA domains. The PA tamine, the latter being in agreement with the substrate pref- domains contact each other directly and bind also to the erence of SBT3 (10). P2-Lys establishes a salt bridge interaction subtilisin domain of the second monomer. This interaction is with the side-chain carboxylate of Asp-213 in pocket S2 (Fig. established via a -hairpin (S9-S10; Fig. S1), which is not S3A). The P3-Glu side chain points in the direction of Arg-318 found in bacterial and mammalian subtilases.
Details
-
File Typepdf
-
Upload Time-
-
Content LanguagesEnglish
-
Upload UserAnonymous/Not logged-in
-
File Pages6 Page
-
File Size-