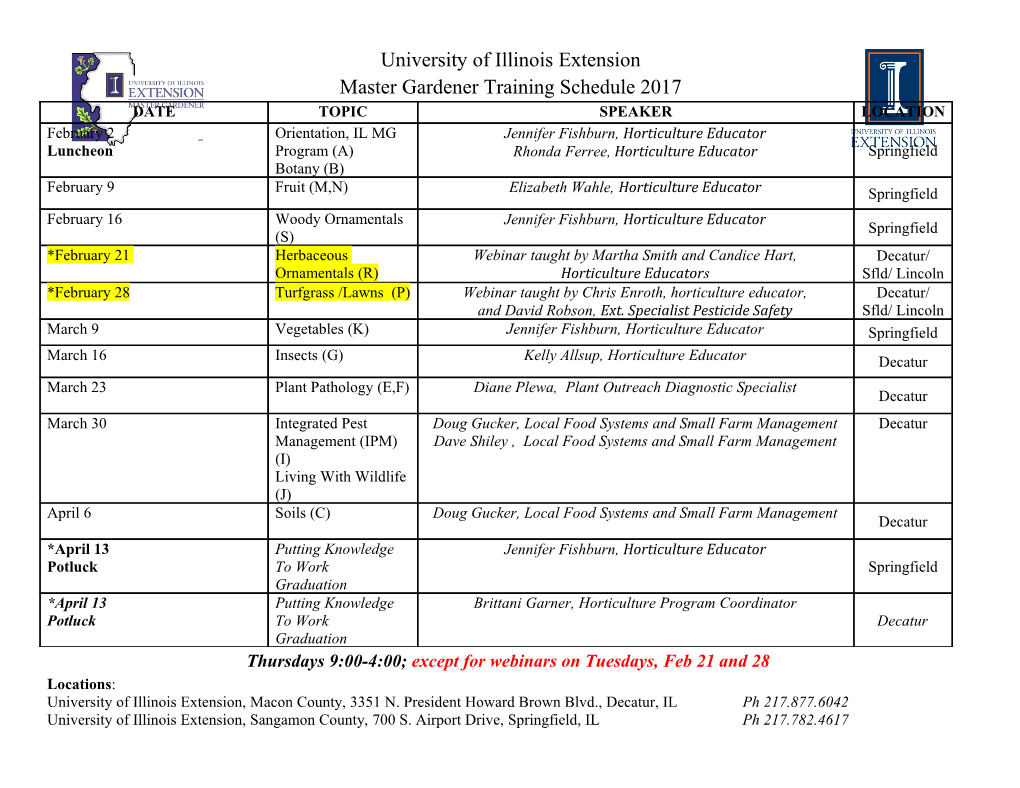
Progress in Materials Science 61 (2014) 216–282 Contents lists available at ScienceDirect Progress in Materials Science journal homepage: www.elsevier.com/locate/pmatsci Pressure-induced amorphization and polyamorphism: Inorganic and biochemical systems ⇑ D. Machon a, , F. Meersman b,c, M.C. Wilding d, M. Wilson e, P.F. McMillan c a Institut Lumière Matière, UMR5306, Université Lyon 1-CNRS, Université de Lyon, 69622 Villeurbanne cedex, France b Department of Chemistry and Materials Chemistry Centre, Christopher Ingold Laboratories, University College London, 20 Gordon Street, London WC1H 0AJ, UK c Department of Chemistry, University of Antwerp, Groenenborgerlaan, 2020 Antwerp, Belgium d Institute of Mathematical and Physical Sciences, Aberystwyth University, Aberystwyth SY23 3BZ, UK e Department of Chemistry, Physical and Theoretical Chemistry Laboratory, University of Oxford, South Parks Road, Oxford OX1 3QZ, UK article info abstract Article history: Pressure-induced amorphization (PIA) is a phenomenon that Received 20 June 2013 involves an abrupt transition between a crystalline material and Received in revised form 2 December 2013 an amorphous solid through application of pressure at tempera- Accepted 3 December 2013 tures well below the melting point or glass transition range. Amor- Available online 14 December 2013 phous states can be produced by PIA for substances that do not normally form glasses by thermal quenching. It was first reported for ice Ih in 1984 following prediction of a metastable melting event associated with the negative initial melting slope observed for that material. The unusual phenomenon attracted intense inter- est and by the early 1990s PIA had been reported to occur among a wide range of elements and compounds. However, with the advent of powerful experimental techniques including high resolution synchrotron X-ray and neutron scattering combined with more precise control over the pressurization environment, closer exam- ination showed that some of the effects previously reported as PIA were likely due to formation of nanocrystals, or even that PIA was completely bypassed during examination of single crystals or materials treated under more hydrostatic compression conditions. Now it is important to understand these results together with related discussions of polyamorphic behavior to gain better under- standing and control over these metastable transformations occur- ring in the low temperature range where structural relaxation and ⇑ Corresponding author. E-mail address: [email protected] (D. Machon). 0079-6425/$ - see front matter Ó 2013 Elsevier Ltd. All rights reserved. http://dx.doi.org/10.1016/j.pmatsci.2013.12.002 D. Machon et al. / Progress in Materials Science 61 (2014) 216–282 217 equilibration processes are severely constrained. The results will lead to useful new high-density amorphous materials or nanocrys- talline composites containing metastable crystalline varieties and the experiments have driven new theoretical approaches to model- ing the phenomena. Here we review the incidence and current understanding of PIA along with related phenomena of density- and entropy-driven liquid–liquid phase transitions (LLPT) and polyamorphism. We extend the discussion to include polymeric macromolecules and biologically-related materials, where the phe- nomena can be correlated with reversible vs irreversible unfolding and other metastable structural transformations. Ó 2013 Elsevier Ltd. All rights reserved. Contents 1. Introduction . ..................................................................... 218 2. Describing the amorphous state . .................................................. 222 2.1. Liquids, glasses and amorphous solids: configurational energy landscape models. 222 2.2. Different routes to amorphous solids. ....................................... 224 3. Methods of investigation . .................................................. 225 3.1. High pressure/high temperature techniques . ....................................... 225 3.2. In situ characterization techniques . ....................................... 226 3.2.1. Energy- vs angle-dispersive synchrotron X-ray and neutron scattering . ....... 226 3.2.2. Vibrational spectroscopy . ............................................. 227 3.2.3. X-ray absorption spectroscopy and EXAFS analysis . .......................... 229 3.2.4. Physical properties measurements . .......................... 230 3.3. Ex situ studies of recovered materials: Transmission electron microcopy and solid state nuclear magnetic resonance . ........................................ 230 3.4. The role of hydrostatic vs non-hydrostatic pressurization . .................... 231 3.5. Computer simulation techniques . ....................................... 232 4. Illustrative examples . .................................................. 233 4.1. H2O: PIA and polyamorphism studies . ....................................... 233 4.1.1. Amorphous states and polyamorphism. .......................... 234 4.1.2. Structural studies of the different amorphous states . .......................... 237 4.1.3. Mechanism and thermodynamic relationships. .......................... 238 4.1.4. Related research work on nanoconfined H2O and water-based solutions . ....... 240 4.2. a-Quartz and other SiO2 polymorphs: PIA and polyamorphism . .................... 241 4.3. Polyamorphism and LLPT: supercooled molten Y2O3/Al2O3 and high pressure liquid phosphorus . ....................................................... 245 4.3.1. Y2O3–Al2O3 system . ............................................. 245 4.3.2. Phosphorus and chalcogen systems . .......................... 248 4.4. Glassy polyamorphism: reversible vs irreversible densification of SiO2 and GeO2 ........... 250 4.4.1. Glassy SiO2: Experimental results . .......................... 250 4.4.2. Liquid and glassy SiO2: Simulation results . .......................... 250 4.4.3. Glassy GeO2: Experimental results . .......................... 251 4.4.4. Liquid and glassy GeO2: Simulation results . .......................... 252 4.4.5. CO2 .................................................................. 253 5. Thermodynamic arguments leading to PIA. ............................... 253 5.1. Metastable transformations . ....................................... 254 5.2. Spinodal limit . ....................................................... 257 5.3. Correlations between PIA, LLPT and polyamorphism . .................... 258 6. Models based on geometric or bonding frustration . ............................... 259 6.1. Steric constraints. ....................................................... 259 6.2. Frustrated phase transition models . ........................................ 260 6.2.1. Ferroelastic glasses . ............................................. 260 6.2.2. Polyamorphism in triphenyl phosphite: PIA or formation of nanocrystals?. ....... 261 6.3. Kinetically hindered chemical decomposition ....................................... 261 Download English Version: https://daneshyari.com/en/article/1656097 Download Persian Version: https://daneshyari.com/article/1656097 Daneshyari.com.
Details
-
File Typepdf
-
Upload Time-
-
Content LanguagesEnglish
-
Upload UserAnonymous/Not logged-in
-
File Pages3 Page
-
File Size-