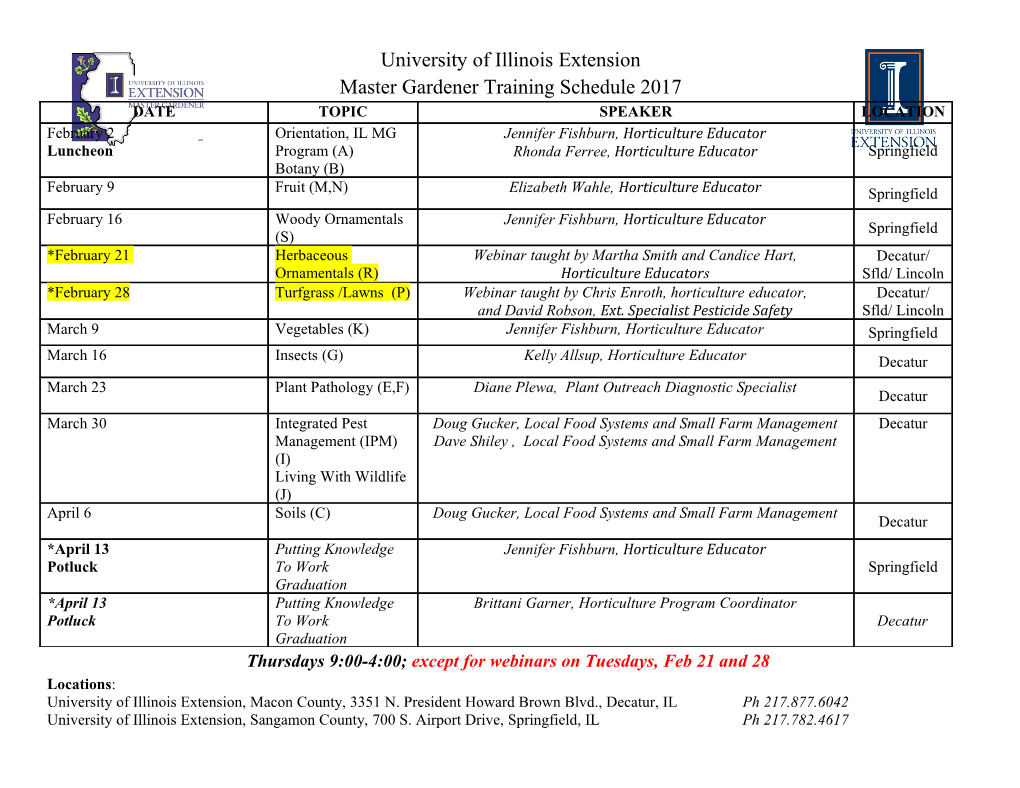
16. THE RESOLUTION OF RIGOR MORTIS Introduction D. E. GOLL IOWA STATE ............................................................................UNIVERSITY The words "rigor" and "mortis" originate from Latin, "rigere", to be stiff, plus "or", more, and from llmortuusI', the past participle of "mori", to die. Thus, rigor mortis may be literally translated as "stiff- ness of death", and correct usage of the term "rigor" must be restricted to those situations involving stiffness, rigidity, or inflexibility. This stiffness or rigidity was frequently noted in corpses of exhausted subjects such as soldiers killed in battle, and the term "rigor mortis" first came into widespread use in connection with forensic medicine. I am emphasizing the close and direct relationship between rigor mortis and stiffness at the outset because, as aptly pointed out at last yeaz's Meat Industry Research Conference by Professor R. E. Davies (15), much of the confusion regarding rigor mortis, and pzrticularly the resolution of rigor mortis, has arisen because of failure to generally recognize that rigor has two closely re- lated but quite different aspects. These two aspects are: 1) a shortening or contraction of the muscle fiber, and 2) a loss of muscle extensibility. To help clarify my discussion this morning, I wish to further divide the extensibility aspect of rigor into a macroscopic phase and a molecular phase. The macroscopic phase of extensibility can be directly observed and measured as ability of a muscle fiber or a bundle of muscle fibers to stretch under the influence of a given weight or force. The molecular phase of extensibility, on the other hand, refers to the ability of actin and myosin filaments in a single sarcomere to slide past one another. When the actin and myosin filaments in a single sarcomere interact, i.e., when actomyosin is formed, molecular inextensibility results in that sarcomere. If a whole series of sarcomeres experience loss of molecular extensibility, then macroscopic inextensibility results. The reason for making this very subtle differentiation between macroscopic and molecular extensibility will become evident later on when I present evidence that molecular inextensi- bility is partially reversible in post-mortem muscle whereas macroscopic measurements of inextensibility do not appear to change during post-mortem storage. In this presentation, I will distinguish between the macroscopic and molecularr phases of rigor only when necessary and hereafter, when I speak of just "extensibility", I will be referring to both the molecular and macroscopic phases. It is now clear that post-mortem muscle always experiences a loss of both macroscopic and molecular extensibility, but under certain condi- tions of temperature, post-mortem shortening or contraction may be so feeble as to escape detection. The thesis I wish to develop in this pre- sentation is that, although molecular inextensibility is a necessary condi- tion for the rigidity or stiffness observed in rigor, neither molecular nor macroscopic inextensibility is itself the primary cause of rigor, i .e., stiffening or hardness, but both are instead more closely related to adenosine triphosphate (A!I'P) depletion in post-mortem muscle. On the other hand, post-mortem shortening or contraction is a direct cause of rigor mortis since attempted shortening by two muscles on opposite sides of a 17. bone would produce a rigidity or stiffening similar to that observed in an isometric contraction. The inextensibility aspect is an essential part of rigor only because the molecular inextensibility condition keeps post- mortem muscle in the shortened state after its ATP supply has beer_ exhausted and it can no longer actively generate tension. If the sarcomeres in post-mortem muscle were not inextensible, they would be free to return to their rest length after the muscle's energy supply had been exhausted, and this would result in loss of the stiffening or rigidity caused by the shortening. Once this clear distinction between the ex- tensibility and shortening aspects of rigor is realized, it becomes neces- sary to talk about resolution of rigor in terms of loss of the ability of post-mortem muscle to maintain its shortened state. I will show later in this presentation that such loss in ability to maintain a shortened or con- tracted condition does in fact occur in post-mortem mxxle, and it is therefore pedagogically correct to talk of a "resolution of rigor." Chemical Changes in Post-Mortem Muscle AS a prelude to detailing the evidence for a resolution of rigor mortis, it will be necessary to review some of the changes which occur in post-mortem muscle and to point out clearly, both in terms of measurement techniques and molecular events, the differences between the extensibility and the shortening aspects of rigor mortis. In the 1800's, the grossly observable stiffness or rigidity associated with rigor mortis was thought to originate from a coagulation of the muscle proteins caused by lactic acid, which was known in those times to appear in post-mortem muscle. Although the lactic acid theory was shown to be untenable already in 1877 by Claude Bernard * s observations that exhausted animals frequently ex- hibited an alkaline rigor, i.e., rigor at muscle pH values near or above neutrality, this lactic acid theory has persisted and can still be found in various mcdif ied forms in some modern textbooks. Bernard ' s observations were later confirmed and extended by Hoet and Marks (27) who, as a result of their studies, postulated that the onset of rigor mortis may be related to disappearance of one of the sugar phosphates. Then in 1943, Erdos (17) showed that the disappearance of ATP was closely related to the onse3 of stiffening in post-mortem muscle and soon afterwards the central role of ATP in rigor mortis was established, largely as a result of the work of E. C. Bate-Smith, J. R. Bendall and their coworkers at Cambridge (1, 2, 3, 4, 5, 6, 7). These early Cambridge studies elucidated many of the rela- tionships among ante-mortem stress, glycogen level, ultimate pH, ATP degradation, and the onset of rigor mortis that are generally accepted yet today. On the basis of these studies, the following chemical changes are now considered to be more or less characteristic of post-mortem muscle: 1) anaerobic breakdown of muscle glycogen to lactic acid, starting imedi- ately after death; 2) a decrease in pH due primarily to the formation of lactic acid; 3) a fall in phosphocreatine content of muscle, this fall occurring very rapidly after muscle glycogen reserves have been exhausted; 4) a decrease in ATP concentration, this decrease occurring very slowly until after the disappearance of phosphocreatine after which time it pro- ceeds rapidly usually to a level less than 20% of its initial level. 1%. Physical Changes in Post-Mortem Muscle The physical changes observed in post-mortem muscle will be rien- tioned only briefly, since this subject has been diseased by the preced- ing speaker. It was quickly recognized by the early investigators that success in rigor mortis research would depend in large part on the avail- ability of an easy and convenient method. for quantitatively and repro- ducibly determining the time of onset of rigor. A kymograph-like apparatus for doing this was developed in 1949 by Bate-Smith and Bendall (4). This apparatus alternately applied a load to a muscle strig for 8 niinutes, and then removed it for 8 minutes after which the cycle w&s repeated until rigor was complete. The extension of the muscle strip caused by applica- tion of the load was recorded, and the muscle was said to be in rigor when application of the load failed to cause any extension of the strip. This apparatus, or the extensibility principle employed by this apparatus (8, 9), has been widely adopted and until recently was almost the only method used for measuring the onset of rigor mortis. With this method, it has been shown that post-mortem muscle extensibility decreases rapidly when the ATP level falls to less than 50$ of its initial level, and that once post- mortem muscle becomes inextensible it does not fully regain its macroscopic extensibility again even though it be stored under aseptic conditions for many days. Thus, using the kymograph-type of instrwnent to measure rigor and defining rigor solely in terms of this measurement, i.e., loss of mac- roscopic extensibility, leads to the conclusion that there is no resolution of rigor mortis. Recently, we have developed an instrument, which we called an "isometer", for determining the onset of rigor mortis (11,29). This in- strument was designed to measure post-mortem tension development in a muscle strip held at a constant length, thereby simulating the conditions to which post-mortem muscle attached to the skeleton --in situ is exposed. The sensitivity and capacity of this method of measuring rigor has been in- creased through the use of isometric myographs in conjunction with an E & M Physiograph. A major improvement in versatility of the isometric measure- ment of rigor was made when it was realized that mmcle strips immersed in mammalian Ringer's solution exhibited the same patterns of tension develop- ment as strips in air. Immersing the strips in solution eliminates spur- ious tension patterns due to drying of the strips in air and also affords the opportunity to control and study the effects of pH, ionic environment, and the addition of fresh ATP, sulfhydryl reagents, etc. on the tension development of post-mortem muscle. This new apparatus is shown in Fig. 1, 2, and 3. It is obvious that the isometric tension measurement of rigor mortis is a direct measure first of the shortening tendency of post-mortem muscle, and then subsequently of the ability of the muscle to maintain its shortened state.
Details
-
File Typepdf
-
Upload Time-
-
Content LanguagesEnglish
-
Upload UserAnonymous/Not logged-in
-
File Pages31 Page
-
File Size-