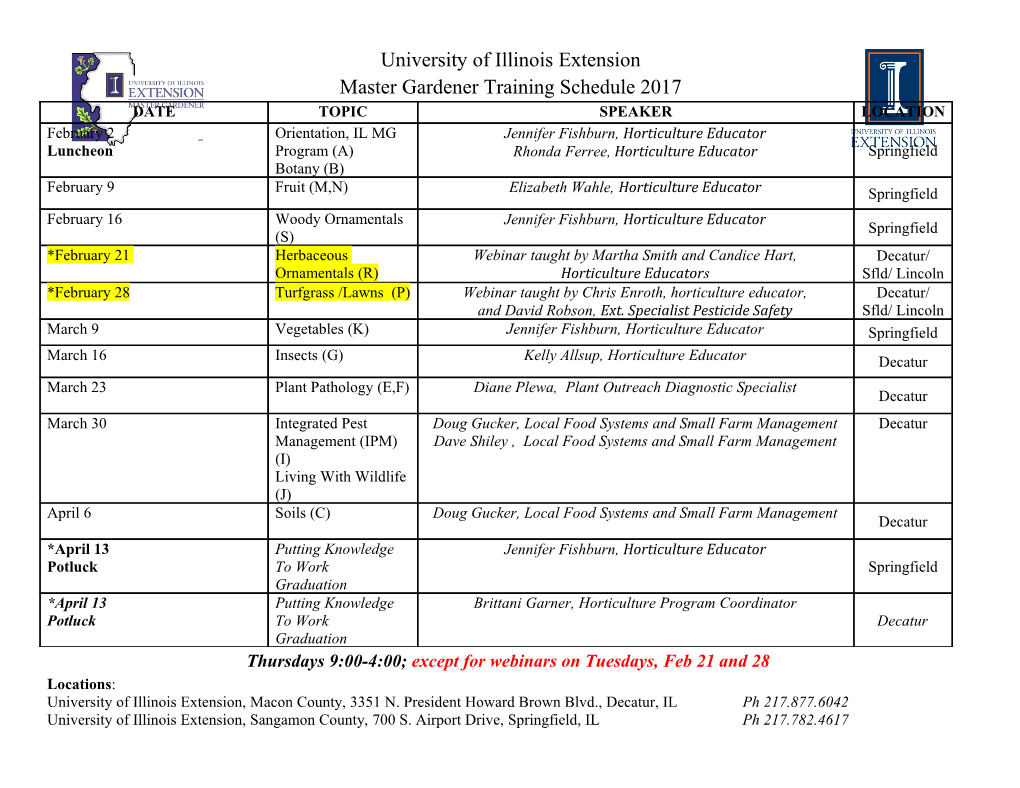
Journal of Oceanography, Vol. 66, pp. 1 to 12, 2010 Review Linking Bacterial Community Structure to Carbon Fluxes in Marine Environments 1 2 TAICHI YOKOKAWA * and TOSHI NAGATA 1Department of Biological Oceanography, Royal Netherlands Institute for Sea Research (NIOZ), P.O. Box 59, 1790 AB, Den Brug, The Netherlands 2Marine Biogeochemistry Group, Ocean Research Institute, The University of Tokyo, Minamidai, Nakano-ku, Tokyo 164-8639, Japan (Received 17 February 2009; in revised form 15 June 2009; accepted 8 July 2009) Microbial oceanography is undergoing a dramatic revolution thanks to the rapid de- Keywords: velopment of novel techniques that allow the examination of microbial diversity and ⋅ Bacterial commu- functions via molecular methods, including genomic and metagenomic analyses. Dur- nity, ⋅ ing the past decade, studies have revealed previously unknown and surprisingly di- ⋅ biogeochemical verse bacterial communities in marine waters. These studies have radically changed cycle, ⋅ carbon flux, our understanding of spatiotemporal patterns in marine bacterial community com- ⋅ microbial food position and the distribution of specific genes. However, our knowledge of the role of webs, individual bacterial subgroups in oceanic food webs and biogeochemical cycles re- ⋅ microbial oceanog- mains limited. To embed the internal dynamics of bacterial communities into marine raphy. biogeochemistry models, the characteristic parameters of individual bacterial sub- groups (i.e., growth, mortality, and utilization of dissolved organic matter) must be determined. Here, we survey the approaches used to assess variation in and factors controlling bacterial communities in marine environments, emphasizing the impor- tance of quantitative studies that examine growth and grazing parameters of bacte- rial subgroups. 1. Introduction Studies of the role of bacteria in marine food webs Over the past three decades, several studies have and biogeochemical processes have generally treated het- examined the abundance, biomass, and production rate erotrophic bacterial communities as an homogeneous pool of heterotrophic bacteria in various marine environments (Ducklow, 2000). This “black box” approach has the ad- (Ducklow, 2000; Church, 2008). These studies have re- vantage of simplicity: ecosystem models are simplified vealed that bacteria consume, on average, 50% of pri- when entire bacterial communities are treated as a single mary production in pelagic ecosystems (Azam and functional group (i.e., heterotrophic bacteria). However, Worden, 2004) and play important roles in major their predictive power is compromised when the internal biogeochemical processes, such as nutrient cycling dynamics of heterogeneous components exhibiting dif- (Kirchman, 2000) and organic matter fluxes (Nagata, ferent physiological and ecological traits are significant. 2008). In addition, studies have shown that bacterial To examine the internal dynamics of bacterial communi- biomass is comparable to, or even exceeds, phytoplankton ties and their implications for biogeochemical cycling in biomass in oceanic environments (Fukuda et al., 1998), marine systems, it is important to understand: 1) the with bacteria representing an important trophic link in spatiotemporal variation in individual bacterial subgroups; marine pelagic food webs (Jürgens and Massana, 2008). 2) mechanisms underlying community structure controls; and 3) contributions of individual groups to carbon flow in microbial food webs. In this review we discuss recent progress in quantitative studies of bacterial community structure in marine environments, emphasizing the roles * Corresponding author. E-mail: [email protected] of bottom-up and top-down factors in determining Copyright©The Oceanographic Society of Japan/TERRAPUB/Springer phylogenetic compositions of bacteria. 1 2. Bacterial Community Analysis in Marine Envi- Alphaproteobacteria dominate the not-yet-cultured bac- ronments—Brief Survey teria (Suzuki and DeLong, 2002). Among Traditionally, microbiologists have examined iso- alphaproteobacterial groups, studies suggest that lated bacterial species using the agar plate technique origi- Roseobacter (González and Moran, 1997; Selje et al., nally developed by Robert Koch (1843–1910; Madigan 2004) and the SAR 11 cluster (Morris et al., 2002) are et al., 2000). These studies examine the physiology and ubiquitous in marine environments. Marine genetics of bacterial strains isolated on culture media. alphaproteobacterial species are now being isolated us- However, in marine environments, bacteria that are ing the extinction dilution technique (Rappé et al., 2002; culturable by regular isolation methods represent less than Giovannoni and Stingl, 2007), revealing unique features 1% of total cells counted using epifluorescence that may be linked to their presence in oligotrophic microscopy (Hobbie et al., 1977; Kogure et al., 1979). oceans. For example, the genome size of Pelagibacter Most marine bacteria are still unculturable and have yet ubique HTCC106, a strain of the SAR11 cluster, is only to be tested for taxonomic affiliations and physiological 1.3M base pairs and encodes the smallest number of pre- and biochemical properties (Fuhrman and Hagström, dicted open reading frames known for a free-living mi- 2008). croorganism (Giovannoni et al., 2005). Conceivably, fu- Advances in culture-independent molecular biology ture studies will add new species to the list of the isolates techniques are changing this situation dramatically of dominant marine bacteria, although the vast majority (Giovannoni et al., 1990; DeLong and Karl, 2005; of marine clones and species detected using culture-in- Fuhrman and Hagström, 2008). These new approaches dependent techniques remain to be isolated. In short, tra- directly examine genetic information from environmen- ditional agar plate determinations of bacterial commu- tal samples (environmental clones) after using polymer- nity compositions have greatly underestimated the diver- ase chain reaction (PCR) to amplify target sequences, sity of bacterial communities in marine environments. without isolating or cultivating bacterial species. The The use of culture-independent gene sequencing taxonomic affiliations of these environmental clones are combined with contemporary genomic approaches (e.g., determined on the basis of small subunit rRNA phylogeny, whole-genome shotgun sequencing - Venter et al., 2004; as originally proposed by Carl Woese (Woese, 1987; Pace, pyrosequencing - Dinsdale et al., 2008) has started to re- 1997). Molecular analyses of environmental clones ob- veal novel features of microbial community structure tained from marine environments have revealed many (metagenomics or community genomics - Moran, 2008; previously unknown bacterial species in coastal and oce- DeLong, 2009). A powerful feature of these new tech- anic environments (Fuhrman and Hagström, 2008; niques is the comprehensive genomic information they Fuhrman, 2009). Sogin et al. (2006) used a provide, not only on 16S rRNA phylogeny, but also on pyrosequencing technique to analyze more than 100,000 functional gene repertoires. At Station ALOHA in the PCR amplicons that span the V6 hypervariable region of subtropical North Pacific, DeLong et al. (2006) used ge- the rRNA gene, revealing tremendous numbers of “rare” nomic analyses to examine prokaryotic communities dis- phylotypes that are present in marine bacterial communi- tributed throughout the water column. They suggested that ties. Studies have also found that Archaea are ubiquitous sequence variation in microbial community genes reflects and abundant in marine systems (DeLong, 1992; Fuhrman the vertical zonation of taxonomic groups as well as the et al., 1992), especially in deep waters (Karner et al., metabolic potentials of prokaryote communities in the 2001; Teira et al., 2006) and surface waters of high lati- oceanic water column. Metagenomic analyses have also tude regions, including the Southern (Church et al., 2003) led to the discovery of novel metabolic pathways and and Arctic Oceans (Kirchman et al., 2007). Suzuki and processes, prominent examples being the findings of DeLong (2002) discovered that, among the major proteorhodopsin (Béjà et al., 2000) and ammonium oxi- phylogenetic groups of Bacteria, Proteobacteria repre- dation by Archaea (Venter et al., 2004). We recommend sented 65% of all 16S rRNA genes of marine clones that readers consult Moran (2008) and DeLong (2009) sequenced, whereas Cytophaga-Flavobacteria- for principles, applications, and the exciting development Bacteroides, Gram-positives, and Cyanobacteria repre- of metagenomic approaches in the field of microbial ocea- sented 10%, 4%, and 3%, respectively. A notably large nography. discrepancy exists in the phylogenetic compositions of Proteobacteria between culturable and unculturable bac- 3. Quantitative Determinations of Bacterial Commu- teria (or perhaps more correctly “not-yet-cultured” bac- nity Structure teria; Fuhrman and Hagström, 2008). In the GenBank 16S Although the advancement of culture-independent rRNA gene database (Benson et al., 2002), techniques has substantially improved our knowledge of Gammaproteobacteria are the dominant group in the over- marine bacterial diversity, little is known of the dynam- all distribution of cultured subgroups, whereas ics and regulation of individual bacterial subgroups. A 2 T. Yokokawa and T. Nagata fundamental requirement for the study of bacterial com- Varela et al., 2008), nucleoside tracers (Hamasaki et
Details
-
File Typepdf
-
Upload Time-
-
Content LanguagesEnglish
-
Upload UserAnonymous/Not logged-in
-
File Pages12 Page
-
File Size-