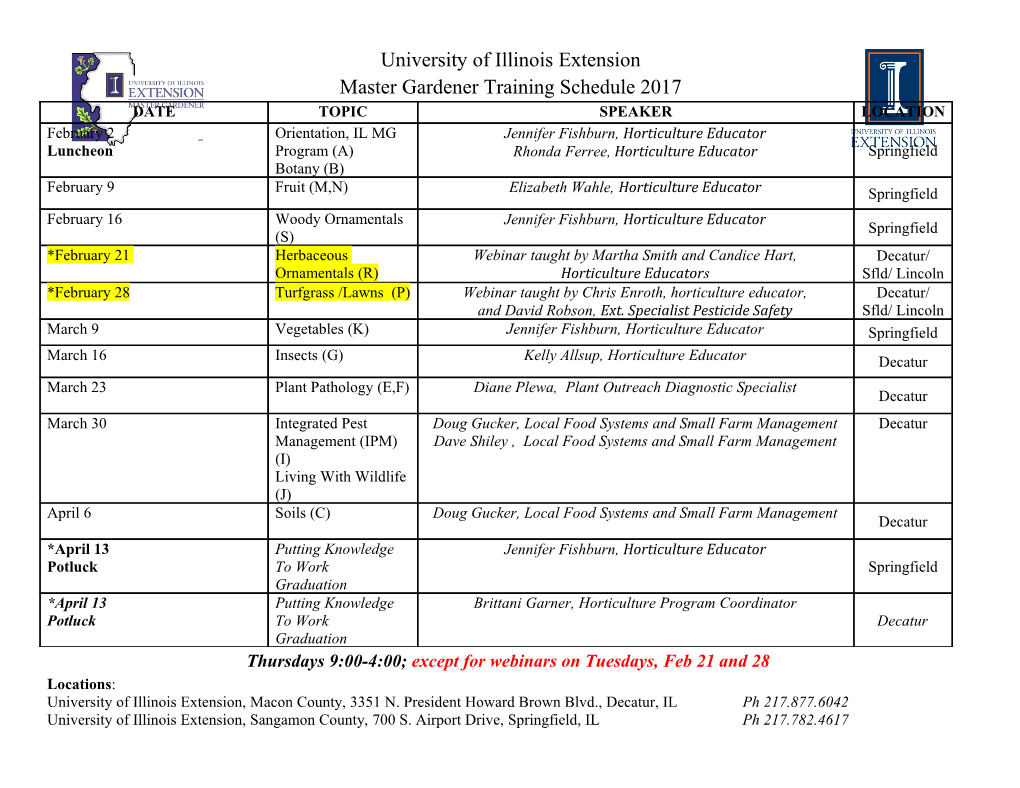
University of Mississippi eGrove Electronic Theses and Dissertations Graduate School 2012 Multi-Configurational Investigation of Thermolytic Pathways of Highly Strained Ring Systems Jeffrey Dwayne Veals Follow this and additional works at: https://egrove.olemiss.edu/etd Part of the Physical Chemistry Commons Recommended Citation Veals, Jeffrey Dwayne, "Multi-Configurational Investigation of Thermolytic Pathways of Highly Strained Ring Systems" (2012). Electronic Theses and Dissertations. 292. https://egrove.olemiss.edu/etd/292 This Dissertation is brought to you for free and open access by the Graduate School at eGrove. It has been accepted for inclusion in Electronic Theses and Dissertations by an authorized administrator of eGrove. For more information, please contact [email protected]. MULTI-CONFIGURATIONAL INVESTIGATION OF THERMOLYTIC PATHWAYS OF HIGHLY STRAINED RING SYSTEMS JEFFREY D. VEALS A dissertation submitted in partial fulfillment of the requirements for the degree of Doctor of Philosophy Chemistry University of Mississippi AUGUST 2012 Copyright c AUGUST 2012 by JEFFREY D. VEALS All rights reserved. ABSTRACT The isomerization pathways of model high energy structures are of interest because of their relation to high energy density fuels. Electron resonance has been found to greatly affect the relative activation barriers for several isomerization pathways, and the major goal of this research was to accurately describe its role in determining the relative barriers for strain energy release pathways. This research was centered around the potential energy surfaces (PESs) for σ bond breaking and π bond rotation in these highly strained structures. Of particular interest was how resonance and or electronegativity would affect the allowed or disallowed nature of the activation barriers in these systems. The two model systems of benzvalene and dihydrobenzvalene have been explored previously. These tricyclic structures were shown to be able to undergo a pericyclic ring opening mechanism. That particular process can either occur in either a conrotatory manner or a disrotatory manner. The disrotatory process was found to be Woodward-Hoffman symmetry allowed in benzvalene, but the conrotatory process was found to be Woodward-Hoffman symmetry allowed in di- hydrobenzvalene. The only difference between these two structures was the presence or absence of a π bond. Much of the current work was focused on deliberately manipulating the allowed and disallowed nature of these type of pericyclic reactions in an attempt to see if the line that is drawn between the allowed and disallowed processes of a particular molecular system could be \blurred". It was also interesting to see that in certain extreme ii cases such as the thermal isomerization of 3,4-diaza-diium-dihydrobenzvalene to 1,2-diaza- diium-dihydropyridazine the activation barriers (disrotatory and conrotatory) were nearly isoenergetic with each other (70.9 kcal/mol vs. 67.2 kcal/mol). In one particular thermal isomerization pathway of 3-aza-benzvalene to pyridine the the allowed and disallowed bar- riers were found to have actually reversed with TSconB having an activation barrier of 38.2 kcal/mol while that of TSdisB was found to be 39.7 kcal/mol at the MRMP/cc-pVTZ level of theory. The PES for π bond rotation was explored in more detail for several trans pyrans and pyridines and also for trans cyclohexene and several of its functional isomers. The ground state geometries for each structure were computed using the multi-configurational self con- sistent field method (MCSCF) with a cc-pVDZ basis set, while energies were computed using multi-reference M¨oller-Plesset second order perturbation theory (MRMP2) with a cc-pVTZ basis set. A multiconfigurational wavefunction was necessary in order to properly describe the σ bond breaking processes as well as the -bond breaking and reforming processes that occurred during the isomerization pathways under study. For the minima on the PESs, energies were also calculated using either the quadratic configuration interaction singles double with iterative triples (QCISD(T) ) method or the coupled cluster singles doubles with iterative triples (CCSD(T)) method with the cc-pVTZ basis set. iii DEDICATION I would like to dedication this work to my loving wife, Javonda. She has put up with and tolerated the long nights of study that were sometimes necessary to make it through this journey. She has been there to help me pick my head up and persevere. To my two wonderful kids - Jaden and Jade - just to see their faces helped me to fight, scratch, and claw to continue until the end. I would also like to thank my parents. Without them none of this would have been possible. I am grateful to my mother because she has always been there for me showing just how proud she been of everything that I have accomplished. I am also grateful to my father for showing me early on what hard work is all about. Thanks for staying on me when I could have easily veered off down the wrong path. Thanks to all of my big siblings: Dennis, Dave Jr., Steve, Beth, and Tim for being there for me. I would like to thank my in-laws for all of their support. All of my late grandparents, particular momma Katie Veals and my "papa" Vernon Matthews, Sr., I know that they would have been so proud of this achievement. Finally, I would like to acknowledge my late uncles Percy Veals, Sr and Jesse "block" Veals, Jr. iv ACKNOWLEDGMENTS First, I would like to thank my advisor, Dr. Steven R. Davis. He took a chance on me and gave me a shot at this thing called research. He has really been supportive, encouraging, and an all-around good role-model. Also, I would like to thank my committee members Dr. Murrell Godfrey, Dr. Nathan Hammer, Dr. Gregory Tschumper, and Dr. Peter Sukanek for their helpful comments, scholarly insight, and demand of excellence. I would like to thank Dr. Walter Cleland, Dr. Maurice Eftink, and Dr. Donald Cole for their support. I would also like to thank Ms. Harriet Hearn, Dr. Safu Aboaku, and Dr. Susan Pedigo for all of their help and support. Finally, I would like to thank my fellow graduate students Dr. Kari Copeland, Margo Montgomery, Gary Brown, Shana Stoddard, Dr. Debra Jo Scardino who have provided invaluable support throughout this process. I would also like to thank Dr. Zhendong Zhao, my former lab mate, for all of his assistance with computer problems. v TABLE OF CONTENTS ABSTRACT ii DEDICATION iv ACKNOWLEDGMENTS v LIST OF FIGURES ix LIST OF TABLES xiii 1 INTRODUCTION 1 1.1 Bicyclobutane . .1 1.2 Tricyclo[3.1.0.02;6]hexane . .5 1.3 Tricyclo[4.1.0.02;7]heptene . .7 1.4 Benzvalene . .8 1.4.1 Research Impetus . .9 2 THEORETICAL BACKGROUND 17 2.1 Theory . 17 2.1.1 Born-Oppenheimer Aproximation . 18 2.1.2 Basis Sets . 20 2.1.3 The Hartree-Fock Approximation . 24 vi 2.1.4 Correlation Methods . 27 2.2 Natural bond orbital analysis . 45 2.3 Computational Details . 48 3 THE STUDY OF 3-AZA-DIHYDROBENZVALENE AND 3,4-DIAZA- DIHYDROBENZVALENE 51 3.1 Introduction . 51 3.2 Computational Methods . 52 3.3 Results and Discussion . 55 3.3.1 3-Aza-dihydrobenzvalene . 55 3.3.2 3,4-Diazatricyclo[3.1.0.02;6]hexane . 75 3.4 Conclusions . 86 3.5 Conclusions . 86 4 THE STUDY OF 3-AZA-BENZVALENE AND 3,4-DIAZA-BENZVALENE 89 4.1 Introduction . 89 4.2 Computational Methods . 91 4.3 Results and Discussion . 92 4.3.1 3-Diazabenzvalene ! Pyridine . 92 4.4 3,4-diaza-benzvalene ! Pyridazine . 105 4.5 Conclusions . 110 5 THE STUDY OF 3-AZA-TRICYCLO[3.1.0.02;6]HEXAN-3-IUM 113 5.1 Introduction . 113 5.2 Computational Methods . 113 5.3 Results and Discussion . 116 5.4 Conclusions . 129 vii 6 THE STUDY OF 3,4-DIAZA-TRICYCLO[3.1.0.02;6]HEXANE-3,4-DIIUM130 6.1 Introduction . 130 6.2 Computational Methods . 130 6.3 Results and Discussion . 132 6.4 Conclusions . 138 7 ISOMERIZATION BARRIERS AND STRAIN ENERGIES OF SELECTED DIHYDROPYRIDINES AND PYRANS WITH TRANS DOUBLE BONDS140 7.1 Introduction . 140 7.2 Computational Methods . 142 7.3 Results and Discussion . 144 7.4 Summary and Conclusions . 155 8 THE STUDY OF ACTIVE SPACE EFFECTS ON THE TRANS TO CIS ISOMERIZATION PROCESS OF OF CYCLOHEXENE AND SEVERAL FUNCTIONAL ISOMERS 158 8.1 Introduction . 158 8.2 Computational Methods . 160 8.3 Results and Discussion . 162 8.4 Conclusions . 179 BIBLIOGRAPHY 183 VITA 194 viii LIST OF FIGURES 1.1 Isomerization processes of bicyclobutane . .2 1.2 Conrotatory vs. disrotatory bond breaking/bond formation. .3 1.3 Isomerization processes of tricyclo[3.1.0.02;6]hexane . .6 1.4 Isomerization processes of tricyclo[4.1.0.02;7]heptene . .8 1.5 Structures to be explored . 11 1.6 Several dihydropyridines and pyrans . 13 1.7 Cyclohexene and several structures of interest . 16 3.1 3-Aza-tricyclo[3.1.0.02;6]hexane. 55 3.2 Conrotatory transition states. 56 3.3 Conrotatory IRC profiles. The chart shows how the conrotatory pathways com- pare to each other. The reaction coordinate has units of amu1=2 bohr. 62 3.4 Disrotatory IRC profiles. The chart shows how the disrotatory pathways compare to each other. The reaction coordinate has units of amu1=2 bohr. 63 3.5 Progression of the C1-C2 σ bond along the IRC. The reaction coordinate has units of amu1=2 bohr. 64 3.6 E,Z -intermediate structures . 66 3.7 Disrotatory transition states. 71 3.8 3,4-Diaza-tricyclo[3.1.0.02;6]hexane. 75 ix 3.9 Path conA and disA for the isomerization of 3,4-diaza-dhb.
Details
-
File Typepdf
-
Upload Time-
-
Content LanguagesEnglish
-
Upload UserAnonymous/Not logged-in
-
File Pages211 Page
-
File Size-