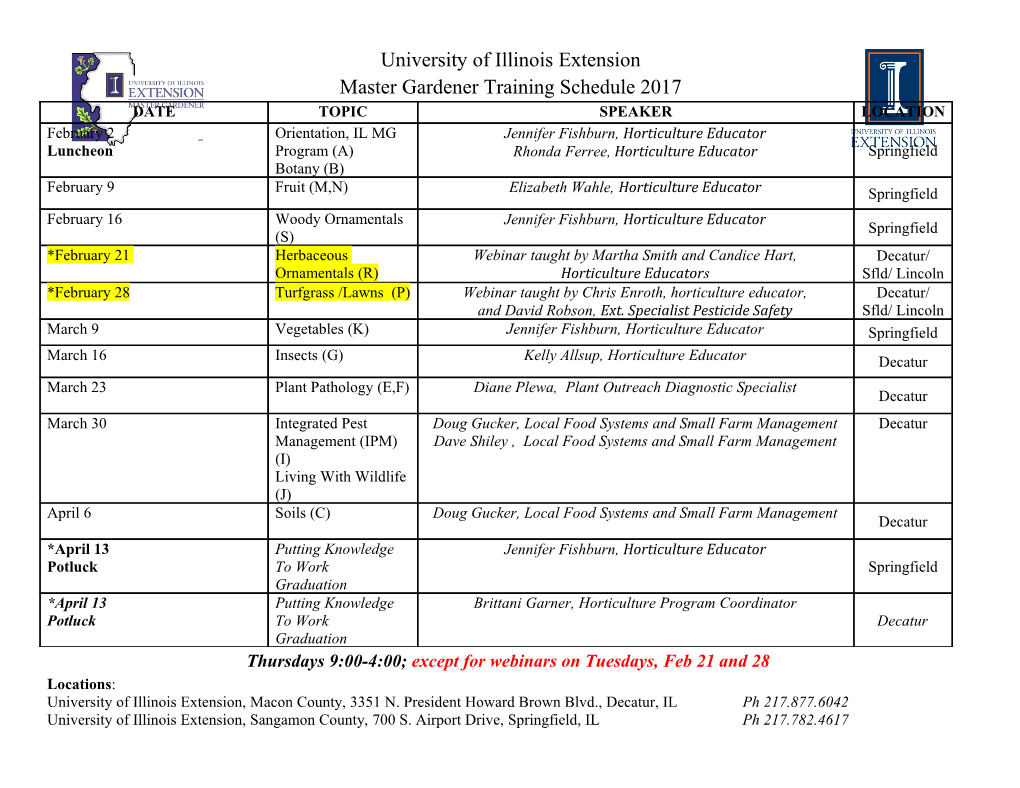
PC67CH04-Herz ARI 11 May 2016 15:17 ANNUAL REVIEWS Further Click here to view this article's online features: • Download figures as PPT slides • Navigate linked references • Download citations Charge-Carrier Dynamics • Explore related articles • Search keywords in Organic-Inorganic Metal Halide Perovskites Laura M. Herz Department of Physics, University of Oxford, OX1 3PU Oxford, United Kingdom; email: [email protected] Annu. Rev. Phys. Chem. 2016. 67:65–89 Keywords First published online as a Review in Advance on photovoltaic, excitons, free charge carriers, charge-carrier recombination, February 26, 2016 bimolecular, Auger, traps The Annual Review of Physical Chemistry is online at physchem.annualreviews.org Abstract This article’s doi: Hybrid organic-inorganic metal halide perovskites have recently emerged as 10.1146/annurev-physchem-040215-112222 exciting new light-harvesting and charge-transporting materials for efficient Copyright c 2016 by Annual Reviews. photovoltaic devices. Yet knowledge of the nature of the photogenerated All rights reserved excitations and their subsequent dynamics is only just emerging. This arti- cle reviews the current state of the field, focusing first on a description of Annu. Rev. Phys. Chem. 2016.67:65-89. Downloaded from www.annualreviews.org the crystal and electronic band structure that give rise to the strong opti- cal transitions that enable light harvesting. An overview is presented of the Access provided by University of Oxford - Bodleian Library on 05/27/16. For personal use only. numerous experimental approaches toward determining values for exciton binding energies, which appear to be small (a few milli-electron volts to a few tens of milli-electron volts) and depend significantly on temperature because of associated changes in the dielectric function. Experimental evidence for charge-carrier relaxation dynamics within the first few picoseconds after ex- citation is discussed in terms of thermalization, cooling, and many-body effects. Charge-carrier recombination mechanisms are reviewed, encom- passing trap-assisted nonradiative recombination that is highly specific to processing conditions, radiative bimolecular (electron-hole) recombination, and nonradiative many-body (Auger) mechanisms. 65 PC67CH04-Herz ARI 11 May 2016 15:17 1. INTRODUCTION Photovoltaic cells incorporating hybrid organic-inorganic metal halide perovskites have seen a phenomenally rapid rise in power conversion efficiencies (PCE) over the past 5 years (1). Following initial demonstrations of perovskite-sensitized liquid-electrolyte solar cells (2), the development of all-solid-state device architectures in 2012 triggered a fast rise in PCE toward 10% (3, 4). These initial studies were based on methylammonium lead tri-iodide, which rapidly became the most commonly investigated material. Optimization of processing conditions, material design, and device architecture led to further rapid improvements (5–8), with PCEs of over 20% reported in 2015 (1, 9). Figure 1c illustrates two of the most commonly implemented device architectures: the dye-sensitized solar cell (DSSC) and the planar heterojunction concept. In the DSSC arrangement, light is absorbed in the perovskite sensitizer acting as the “dye,” after which electrons may transfer into the mesoporous TiO2 and holes into the hole-transporting material (HTM) (4, 10). In the simplified planar heterojunction architecture (6), electron-hole pairs generated in the perovskite absorber must diffuse to the selective HTM and electron-transporting material layers, requiring sufficiently high charge-carrier diffusion lengths. In a third concept, the meso-superstructured configuration, an insulating mesoporous Al2O3 scaffold aids coating uniformity (3, 5). Following the initial success of these materials as active ingredients in photovoltaics, a parallel strand of research soon emerged, focusing on light-emitting diodes (11, 12) and amplified spontaneous emission or lasing (13–17). Although applications based on these materials have hence progressed a Perovskite ABX3 b c Solar cell device architectures DSSC Metal cathode HTM Perovskite MMesoporousesoporous TTiOiO2 ETM Metal anode Planar heterojunction Metal cathode HTM c A: Organic cation B: Metal cation Perovskite Annu. Rev. Phys. Chem. 2016.67:65-89. Downloaded from www.annualreviews.org b X: Halide anion a ETM Access provided by University of Oxford - Bodleian Library on 05/27/16. For personal use only. Metal anode Figure 1 (a) Perovskite unit cell of the pseudocubic lattice showing the organic A cation (e.g., methylammonium, formamidinium) in orange, the + + − − − metal B cation (e.g., Pb2 or Sn2 ) in blue, and the X anion (e.g., Cl ,Br ,orI )ingreen.(b) Representation of the perovskite lattice 4− in terms of an anion corner-shared 3D network of (BX6) octahedra, with B cations at their centers and A cations between them. Octahedral tilt combinations result in the adopted crystal symmetry space group. (c) Schematic depiction of two typical device architectures commonly used for perovskite solar cells: the dye-sensitized solar cell (DSSC) arrangement and the simplified planar heterojunction architecture. Typical materials used in high-performing photovoltaics cells are as follows: a nontransparent electrode (silver or gold), a transparent electrode (e.g., fluorine tin oxide), a hole-transporting material (HTM; spiro-OMeTAD or PEDOT:PSS), and an electron-transporting material (ETM; compact TiO2 or PCBM) (18). A transparent substrate (e.g., glass) is situated below (above) the anode (cathode) in the normal (inverted) configuration to provide support. 66 Herz PC67CH04-Herz ARI 11 May 2016 15:17 at an amazing speed, an understanding of the fundamental photophysics enabling such success is only just emerging. Yet such knowledge is critical for the design of high-performance materials because the generation, relaxation, and recombination of photoexcited charge species have a direct bearing on how effectively light is converted into electrical current, and vice versa. This review summarizes the current state of the field, providing a detailed summary of ongoing research elucidating the fundamental nature of photoexcited states and their dynamics in hybrid metal halide perovskites. Strictly speaking, perovskite is the calcium titanium oxide mineral CaTiO3 named after the Russian mineralogist Lev Perovski. Compounds of the same crystal structure type are said to assume a perovskite structure (19) as shown in Figure 1a,b with A cations (organic cations) in the corners, the B cation (metal cation) at the center, and the X anion (halide anion) centered on the faces of the unit cell. Goldschmidt (19) proposed in 1926 that a solid will only assume the , + = perovskite√ structure if the respective ionic radii RA, RB and RX fulfill the relation RA RX t × 2(RB + RX), with the tolerance factor t ranging between 0.8 and 1. A large number of materials exist within the perovskite structure (20, 21); however, highly efficient photovoltaic cells using such perovskite materials as light absorbers have so far been demonstrated only for X anions − − − composing halogens such as I ,Br ,andCl . Within the stoichiometry ABX3, the halogen anion needs to be counterbalanced by a monovalent and a divalent A and B species for overall charge neutrality. In fully inorganic metal halide perovskites, the monovalent metal may, for example, be an alkali such as Cs+ and the divalent species Pb2+,Sn2+,orGe2+. In hybrid organic-inorganic halide perovskites, which are the highest-performing active materials in photovoltaic cells to date, the monovalent metal is replaced by an organic cation of single positive charge. The most + commonly used organic A ion is methylammonium (MA), CH3NH3 (3), but formamidinium (FA), + H2NCHNH2 (22), and mixed systems (9) are also emerging with excellent results. The divalent metal (B cation) utilized in the best performing active materials for such perovskite photovoltaics has almost exclusively been lead (Pb2+), which has the additional benefit of being an abundant material in Earth’s ore (23). However, concerns about lead’s toxicity have triggered a search for alternative B cations. The tin-based perovskite MASnI3 has been demonstrated to allow for photovoltaic PCEs of approximately 6% (24, 25), which is respectable but still lower than those reported for their lead-containing counterparts [now exceeding 20% (1)]. This review predominantly focuses on hybrid organic-inorganic halide perovskite materials that have shown particular promise for photovoltaic applications, that is, materials for which A is either the MA or FA cation, B is Pb2+ unless stated otherwise, and X is the halogen an- − − − ion I ,Br ,orCl or a mixture thereof. The mixed halide perovskite material MAPbBry I3−y (0 ≤ y ≤ 3) exhibits a band gap that tunes continuously between ∼1.5 eV and ∼2.2 eV with an Annu. Rev. Phys. Chem. 2016.67:65-89. Downloaded from www.annualreviews.org increasing bromide fraction y/3 (26), making this system attractive for tandem cells, for example. However, the apparent chloride equivalent MAPbClx I3−x showed no evidence for band-gap shifts Access provided by University of Oxford - Bodleian Library on 05/27/16. For personal use only. when chlorine was included in the solution precursor materials (3). It should be stressed that this material is generally produced from the organic and inorganic precursors MAI and PbCl2 mixed in the ratio 3:1; therefore, no inclusion of chloride is in principle required for the formation of the perovskite structure,
Details
-
File Typepdf
-
Upload Time-
-
Content LanguagesEnglish
-
Upload UserAnonymous/Not logged-in
-
File Pages28 Page
-
File Size-