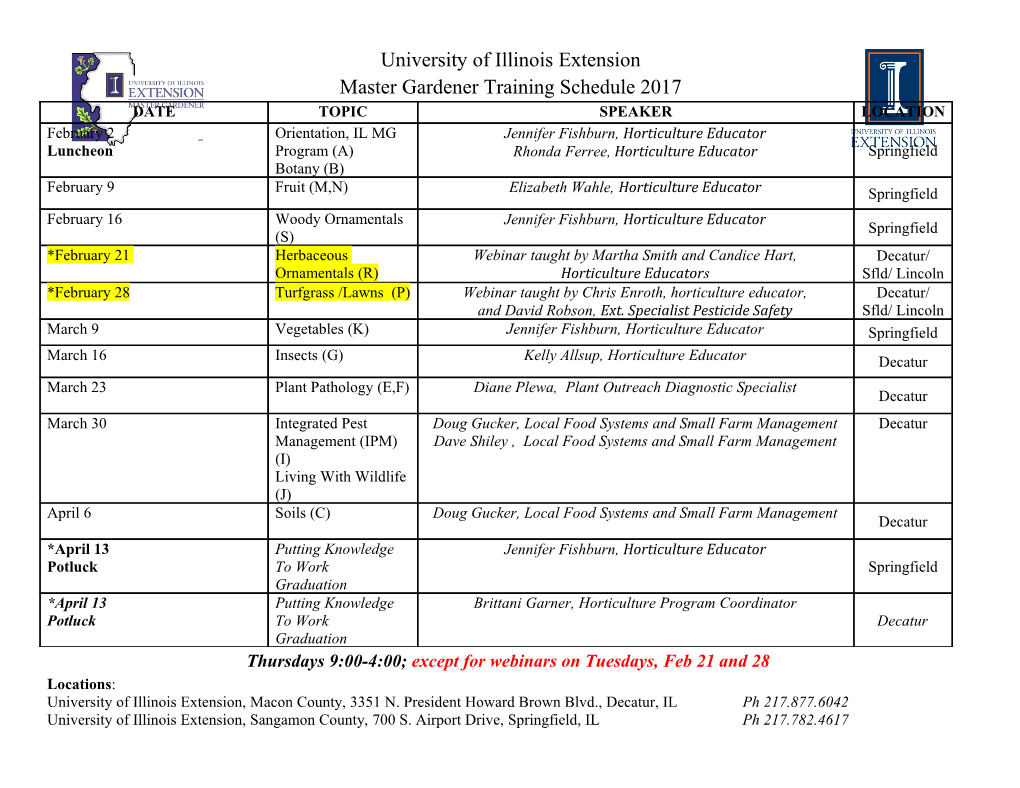
CELT: A Low-latency, High-quality Audio Codec Dr. Jean-Marc Valin, Gregory Maxwell, and Dr. Timothy B. Terriberry The Xiph.Org Foundation Outline ● Introduction and Motivation ● CELT Design ● libcelt ● Demo ● Conclusion 2 The Xiph.Org Foundation Introduction ● Two common types of lossy audio codecs – Speech/communication (G.72x, GSM, AMR, Speex) ● Low delay (15-30 ms) ● Low sampling rate (8 kHz to 16 kHz): limited fidelity ● No support for music – General purpose (MP3, AAC, Vorbis) ● High delay (> 100 ms) ● High sampling rates (44.1 kHz or higher) ● "CD-quality" music – We want both: high fidelity with very low delay 3 The Xiph.Org Foundation Introduction ● Low delay is critical to live interaction – Prevents collisions during conversation – Reduce need for echo cancellation ● Good for small, embedded devices without much CPU – High delay Low delay Higher sense of presence (~250 ms) (~15 ms) – Allows synchronization for live music ● Need less than 25 ms total delay to synchronize (Carôt 2006) ● Equivalent to sitting 8 m apart (farther requires a conductor) ● Lower delay in the codec increases range – 1 ms = 200 km in fiber 4 The Xiph.Org Foundation Introduction ● No entrenched standard in this space – G.722.1C (ITU-T) [40 ms delay, up to 32 kHz] – AAC-LD (MPEG) [20-50 ms delay, up to 48 kHz] – ULD (Fraunhofer) [< 10 ms delay, up to 48 kHz] ● CELT is already ahead of the competition – Delay: Configurable, 1.3 ms to 24 ms, ~8 ms typical – Quality (at equivalent rates): Much better than G.722.1C, as good as or better than AAC-LD, better than ULD – Flexibility: 24 kbps to 160+ kbps, 32 kHz to 96 kHz, configurable delay, low-complexity mode – Freedom: Open source (BSD), no patents 5 The Xiph.Org Foundation Outline ● Introduction ● CELT Design ● libcelt ● Demo ● Conclusion 6 The Xiph.Org Foundation CELT: "Constrained Energy Lapped Transform" ● Transform codec (MDCT, like MP3, Vorbis) – Short windows (~8 ms) → poor frequency resolution ● Explicitly code energy of each band of the signal – Coarse shape of sound preserved no matter what ● Code remaining details using vector quantization ● Also uses pitch prediction with a time offset – Similar to linear prediction used by speech codecs – Helps compensate for poor frequency resolution 7 The Xiph.Org Foundation Outline ● Introduction ● CELT Design – "Lapped Transform" – "Constrained Energy" – Coding Band Shape – Performance Tests ● libcelt ● Demo ● Conclusion 8 The Xiph.Org Foundation "Lapped Transform" Time-Frequency Duality ● Any signal can be represented as a weighted sum of cosine curves with different frequencies ● The Discrete Cosine Transform (DCT) computes the weights for each frequency 220 Hz (A3) 440 Hz (A4) 1245 Hz (D#5) 9 The Xiph.Org Foundation "Lapped Transform" Discrete Cosine Transform ● The "Discrete" in DCT means we're restricted to a finite number of frequencies – As the transform size gets smaller, energy "leaks" into nearby frequencies (harder to compress) N=48000 10 The Xiph.Org Foundation "Lapped Transform" Discrete Cosine Transform ● The "Discrete" in DCT means we're restricted to a finite number of frequencies – As the transform size gets smaller, energy "leaks" into nearby frequencies (harder to compress) N=4096 (Maximum Vorbis block size) 11 The Xiph.Org Foundation "Lapped Transform" Discrete Cosine Transform ● The "Discrete" in DCT means we're restricted to a finite number of frequencies – As the transform size gets smaller, energy "leaks" into nearby frequencies (harder to compress) N=1024 (Typical Vorbis block size) 12 The Xiph.Org Foundation "Lapped Transform" Discrete Cosine Transform ● The "Discrete" in DCT means we're restricted to a finite number of frequencies – As the transform size gets smaller, energy "leaks" into nearby frequencies (harder to compress) N=256 (CELT can use 64...512) 13 The Xiph.Org Foundation "Lapped Transform" Discrete Cosine Transform ● The "Discrete" in DCT means we're restricted to a finite number of frequencies – As the transform size gets smaller, energy "leaks" into nearby frequencies (unstable over time) N=256 (CELT can use 64...512) Frame 2... 14 The Xiph.Org Foundation "Lapped Transform" Discrete Cosine Transform ● The "Discrete" in DCT means we're restricted to a finite number of frequencies – As the transform size gets smaller, energy "leaks" into nearby frequencies (unstable over time) N=256 (CELT can use 64...512) Frame 3... 15 The Xiph.Org Foundation "Lapped Transform" Modified DCT ● The normal DCT causes coding artifacts (sharp discontinuities) between blocks, easily audible ● The "Modified" DCT (MDCT) uses a decaying window to overlap multiple blocks – Same transform used in MP3, Vorbis, AAC, etc. – But with much smaller blocks, less overlap 16 The Xiph.Org Foundation Outline ● Introduction ● CELT Design – "Lapped Transform" – "Constrained Energy" – Coding Band Shape – Performance Tests ● libcelt ● Demo ● Conclusion 17 The Xiph.Org Foundation "Constrained Energy" Critical Bands ● The human ear can hear about 25 distinct "critical bands" in the frequency domain – Psychoacoustic masking within a band is much stronger than between bands Threshold of detection in the presence of masker at 1kHz with a bandwidth of 1 critical band and various levels. Image blatantly stolen from http://www.tonmeister.ca/main/textbook/node331.html 18 The Xiph.Org Foundation "Constrained Energy" Critical Bands ● Group MDCT coefficients into bands approximating the critical bands (Bark scale) – We limit bands to contain at least 3 coefficients to minimize per-band overhead – Insufficient frequency resolution for all the bands – But we spend most of our bits on LFs anyway Bark Scale vs. CELT @ 48kHz, Frame Size=256 Bark CELT 0 2000 4000 6000 8000 10000 12000 14000 16000 18000 20000 Frequency (Hz) 19 The Xiph.Org Foundation "Constrained Energy" Coding Band Energy ● Most important psychoacoustic lesson learned from Vorbis: Preserve the energy in each band ● Vorbis does this implicitly with its "floor curve" ● CELT codes the energy explicitly – Coarse energy (6 dB resolution), predicted from previous frame and from previous band ● Prediction saves 28 bits/frame, 5.6 kbps with 5 ms frames – Fine energy, improves resolution where we have available bits, not predicted 20 The Xiph.Org Foundation "Constrained Energy" Coding Band Energy ● CELT (green) vs original (red) – Notice the quantization between 8.5 kHz and 12 kHz – Speech is intelligible using coarse energy alone (~9 kbps for 5.3 ms frame sizes) 21 The Xiph.Org Foundation Outline ● Introduction ● CELT Design – "Lapped Transform" – "Constrained Energy" – Coding Band Shape – Performance Results ● libcelt ● Demo ● Conclusion 22 The Xiph.Org Foundation Coding Band Shape ● After normalizing, each band is represented by an N-dimensional unit vector – Point on an N-dimensional sphere – Describes "shape" of energy within the band ● Code this shape using two pieces: – An adaptive codebook using previously decoded signal content to predict the current frame – A fixed codebook to handle the part of the signal that can't be predicted (the "innovation") ● Latter uses vector quantization 23 The Xiph.Org Foundation Coding Band Shape Vector Quantization ● Approximates a multidimensional distribution with a finite number of codewords (vectors) Scalar Quantization (2 bits/dim) Vector Quantization (2 bits/dim) RMS error = 0.89 RMS error = 0.71 (20% better) 24 The Xiph.Org Foundation Coding Band Shape Vector Quantization ● Easily scales to less than 1 bit per dimension (very important for HF bands: 50-200 dims) Scalar Quantization (0.5 bits/dim) Vector Quantization (0.5 bits/dim) RMS error = 2.93 RMS error = 1.63 (44% better) 25 The Xiph.Org Foundation Coding Band Shape Algebraic Vector Quantization ● CELT requires a lot of codebooks – Every band can have a different # of dimensions – Exact number of bits available for each band varies from packet to packet ● CELT requires large codebooks – Exponential in # of dimensions: 50 dims at 0.6 bits/ dim. requires over a billion codebook entries – We couldn't even store one codebook that large – And even if we could, it'd take forever to search ● But we have uniformly distributed unit vectors 26 The Xiph.Org Foundation Coding Band Shape Algebraic Vector Quantization ● Use a regularly structured, algebraic codebook: Pyramid Vector Quantization (Fischer, 1986) – We want evenly distributed points on a sphere ● Don't know how to do that for arbitrary dimension – Use evenly distributed points on a pyramid instead ● For N dimensional vector, allocate K "pulses" ● Codebook consists vectors with integer coordinates whose magnitudes sum to K 27 The Xiph.Org Foundation Coding Band Shape Pyramid Vector Quantization ● PVQ codebook has a fast enumeration algorithm – Converts between vector and integer codebook index – O(N+K) (lookup table, muls) or simpler O(NK) (adds) – Latter great for embedded processors, often faster ● Fast codebook search algorithm: O(N·min(N,K)) – Divide by L1 norm, round down: at least K-N pulses – Place remaining pulses (at most N) one at a time ● Codebooks larger than 32 bits – Split the vector in half and code each half separately 28 The Xiph.Org Foundation Coding Band Shape Pitch Prediction ● Short block sizes → poor frequency resolution – Speech/music have periodic, stationary content – Can't represent the period accurately via short MDCT ● Pitch prediction compensates for poor resolution – Search the past 1024 decoded samples in the time domain, code the offset of the best match ● Resolution equal to the sampling rate 48000 48000 ● Range (48 kHz,
Details
-
File Typepdf
-
Upload Time-
-
Content LanguagesEnglish
-
Upload UserAnonymous/Not logged-in
-
File Pages62 Page
-
File Size-