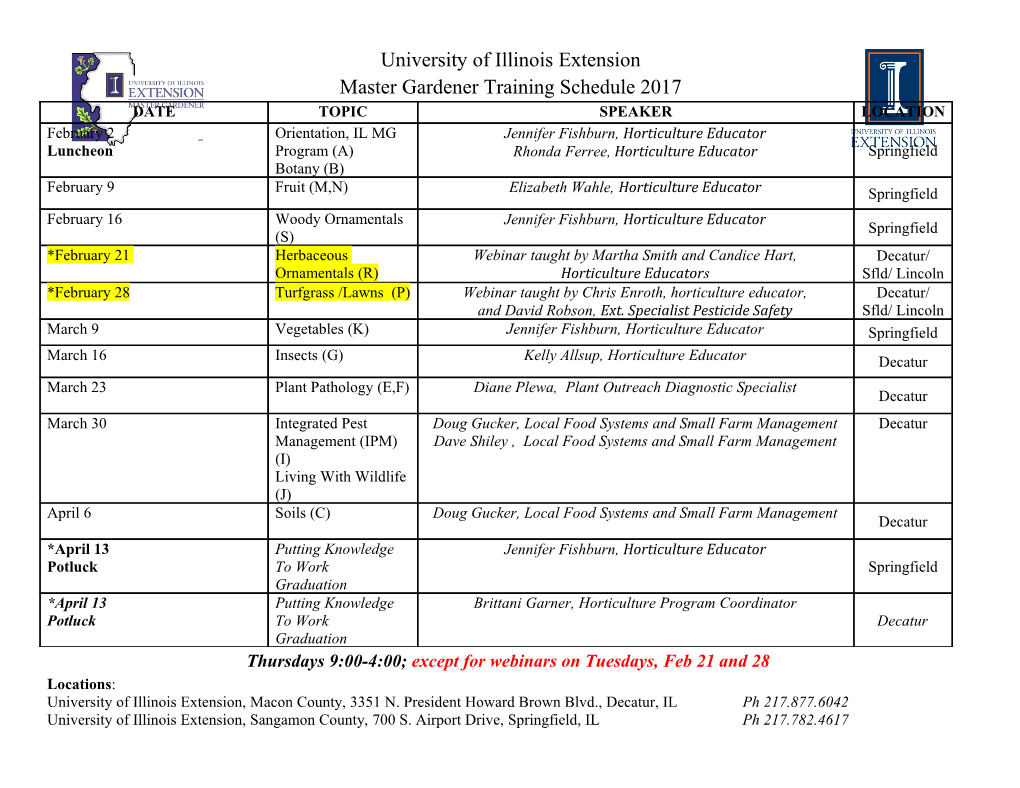
ARTICLE Received 5 Jan 2011 | Accepted 2 Mar 2011 | Published 5 Apr 2011 DOI: 10.1038/ncomms1264 A flow-microreactor approach to protecting-group- free synthesis using organolithium compounds Heejin Kim1, Aiichiro Nagaki1 & Jun-ichi Yoshida1 Protecting-group-free synthesis has received significant recent research interest in the context of ideal synthesis and green sustainable chemistry. In general, organolithium species react with ketones very rapidly, and therefore ketone carbonyl groups should be protected before an organolithium reaction, if they are not involved in the desired transformation. If organolithium chemistry could be free from such a limitation, its power would be greatly enhanced. Here we show that a flow microreactor enables such protecting-group-free organolithium reactions by greatly reducing the residence time (0.003 s or less). Aryllithium species bearing ketone carbonyl groups are generated by iodine–lithium exchange reactions of the corresponding aryl iodides with mesityllithium and are reacted with various electrophiles using a flow-microreactor system. The present method has been successfully applied to the formal synthesis of Pauciflorol F. 1 Department of Synthetic and Biological Chemistry, Graduate School of Engineering, Kyoto University, Nishikyo-ku, Kyoto 615-8510, Japan. Correspondence and requests for materials should be addressed to J.-i.Y. (email: [email protected]). NatURE COMMUNicatiONS | 2:264 | DOI: 10.1038/ncomms1264 | www.nature.com/naturecommunications © 2011 Macmillan Publishers Limited. All rights reserved. ARTICLE NatUre cOMMUNicatiONS | DOI: 10.1038/ncomms1264 ontinuous-flow microreactors based on microfluidics1–3 have various electrophiles using a flow-microreactor system by greatly been recognized as powerful tools for chemical synthesis, reducing the residence time (0.003 s or less). We also show that the and studies on their characteristic features have uncovered present method has been successfully applied to the formal synthe- C 4–25 a rich variety of synthetic applications in recent years . Flow- sis of Pauciflorol F. microreactor synthesis has also received much attention from the viewpoint of synthesis based on reaction integration26–30 and green Results sustainable synthesis31. Such successful applications speak well for Generation and reactions of acyl-substituted aryllithiums. We the power of the flow-microreactor method in chemical synthesis. began our investigation by conducting the iodine–lithium exchange It is important to note that the reaction time in a flow microreac- reaction of o- and p-acyliodobenzenes followed by trapping with tor is defined as the residence time between a reagent inlet and the methanol using the flow-microreactor system, as shown in Figure 1 quencher inlet, which can be controlled precisely and reduced to (see also Supplementary Fig. S1). Mesityllithium was first generated millisecond order by adjusting the length between these positions by a bromine–lithium exchange reaction of 2-bromo-1,3,5- and the flow speed. On the basis of such a feature of flow microreac- trimethylbenzene (mesityl bromide) and n-butyllithium at 0 °C, tors, we have recently proposed the concept of flash chemistry32–35, which enables the use of short-lived highly reactive intermediates a O O O for synthesis. Such intermediates can be rapidly generated and MesLi MeOH transferred to another location in the flow system for use in subse- n-Bu n-Bu n-Bu quent reactions before they decompose. It is also noteworthy that the total production capacity over time of continuous-flow microre- I Li H actors is much greater than may be imagined, although the reactor’s 1 2 3 capacity at any one time is small. Therefore, flow-microreactor syn- 2 thesis can be used not only for laboratory-scale synthesis but also i-Pr for the production of chemical substances on an industrial scale. In fact, there are flow microreactors that fit in the palm of the hand O and can produce several tons of products per year36,37. Armed with an understanding of the essential principles, chemists are now in n-Bu a position to enhance the capability of flow microreactions and to develop new synthetic transformations that are difficult to achieve 4 by conventional batch reactions. 38,39 b c Organolithium species serve as powerful reagents in organic 100 100 synthesis. However, they are not compatible with electrophilic func- 90 90 80 80 tional groups such as ketone carbonyl groups. In fact, organolithium 70 70 ) ) 3 species react with ketones very rapidly. In some cases, organolithium 60 4 60 50 50 species can be generated in the presence of ketones and quenched 40 40 Yield (% 40 Yield (% 30 30 in situ by the ketone carbonyl group . However, if a ketone carbonyl 4 20 3 20 group is not involved in the desired transformation, it should be 10 10 0 0 protected before an organolithium reaction, although ketone car- 0 0.5 1 1.5 2 2.5 3 0 0.005 0.01 0.015 bonyl groups survive in reactions of some less reactive organome- Residence time in R2 (s) Residence time in R2 (s) tallics41–43. Therefore, if organolithium reactions can be conducted without protecting the ketone carbonyl groups, the power of Figure 2 | The effect of residence time in R2 for the reaction of organolithium chemistry will be greatly enhanced. o-pentanoyliodobenzene (1). (a) Reaction of 1 with MesLi to yield Here we show that a flow microreactor enables protecting-group- o-pentanoyl-substituted phenyllithium (2) followed by trapping with free organolithium reactions by greatly reducing the residence time MeOH to produce protonated product 3. Undesired dimerization of 2 gave (0.003 s or less); aryllithium species bearing ketone carbonyl groups by-product 4. (b) Plots of the yield of 3 and 4 against the residence time in are generated by iodine–lithium exchange reactions of the cor- R2 ( < 3 s). (c) Plots of the yield of 3 and 4 against the residence time in R2 responding aryl iodides with mesityllithium and are reacted with ( < 0.015 s). a O b O R Li R I R2 R2 M3 MesBr M2 E: electrophile R1 M1 R3 M2 n-BuLi 0 °C O –70 °C M3 E R Figure 1 | A flow-microreactor system. (a) A schematic diagram of the system. 2-Bromo-1,3,5-trimethylbenzene (mesityl bromide, MesBr) was reacted with n-butyllithium using T-shaped micromixer M1 and microtube reactor R1 at 0 °C. The resulting mesityllithium solution was reacted with an acyliodobenzene in T-shaped micromixer M2 and microtube reactor R2 at –70 °C. The resulting short-lived acylphenyllithium species was trapped with an electrophile using T-shaped micromixer M3 and microtube reactor R3 at − 70 °C. (b) An integrated device in which M2 (inner diameter: 250 µm), R2 (inner diameter: 250 µm, length: 1.0 cm) and M3 (inner diameter: 250 µm) are combined. NatUre cOMMUNicatiONS | 2:264 | DOI: 10.1038/ncomms1264 | www.nature.com/naturecommunications © 2011 Macmillan Publishers Limited. All rights reserved. NatURE COMMUNicatiONS | DOI: 10.1038/ncomms1264 ARTICLE Table 1 | Generation of acyl-substituted aryllithiums and reactions with electrophiles in a flow-microreactor system. Entry Substrate Electrophile Product Yield* Entry Substrate Electrophile Product Yield* O O O O t-Bu n-Bu n-Bu t-Bu H N 1 MeOH 90 (91†) 13 PhNCO Ph 87 I H I O 1 3 20 21 O O O n-Bu 2 Me3SiOTf 86 (91†) 14 MeOH 78† SiMe3 I H 8 22 23 O O n-Bu 3 Bu3SnCl 86 15 PhCHO HO 73 (75†) SnBu3 Ph 9 24 O O n-Bu 4 MeO2CCl 68 (70†) 16 PhCOMe HO 76 CO2Me Ph Me 10 25 n-Pr O O Ph O Ph H 5 PhCOMe 81 17 PhNCO N 71 Me Ph I Ph 11 26 O 27 n-Pr O O O n-Bu 6 PhCOCy 81 18 n-Bu MeOH 67† Cy H Ph I 12 28 3 HO n-Bu O O n-Bu H 7 PhCHO 60 19 PhNCO N 51 Ph Ph 13 O 29 O O O O Et Et Me Me 8 MeOH 83† 20 MeOH 54 (76‡) I H I H 5 7 14 15 O O Me Et Ph 9 Me3SiOTf 81 (84†) 21 PhCHO 78‡ SiMe3 OH 30 16 O n-Bu n-Bu 10 MeO CCl Et 65 (69†) I S H S 2 22 O MeOH O 74 CO Me 2 31 32 17 O O Ph n-Bu S Me Me HO O 11 MeOH 77† 23 PhCHO 77 I H 33 18 7 H O Ph N n-Bu S Me 12 MeOTf 42† 24 PhNCO O O 59 Me 34 19 THF, tetrahydrofuran. 2 Reaction conditions: acyliodobenzene (0.20 M in THF), MesLi (1.3 equivalent in THF/hexane, generated in situ), electrophile (2.0 equivalent in THF or Et2O), residence time in R =0.003 s. *Isolated yields unless otherwise stated. †GC (gas chromatography) yields obtained using an internal standard. ‡ Residence time in R2=0.0015 s. NatURE COMMUNicatiONS | 2:264 | DOI: 10.1038/ncomms1264 | www.nature.com/naturecommunications © 2011 Macmillan Publishers Limited. All rights reserved. ARTICLE NatUre cOMMUNicatiONS | DOI: 10.1038/ncomms1264 because preliminary studies showed that mesityllithium was the most a O O O MesLi MeOH effective compound for this purpose. The iodine–lithium exchange Me Me Me reaction of an acyliodobenzene using the resulting mesityllithium was conducted at − 70 °C. The short-lived acylphenyllithium I Li H species thus produced was trapped with methanol as an electrophile 5 6 7 at − 70 °C (Fig. 1a). b 80 We focused on the generation of o-pentanoyl-substituted phenyl- 70 lithium (2) generated from o-pentanoyliodobenzene (1). Methanol 60 was used as a quenching electrophile (Fig. 2a). The reactions were ) 50 7 carried out with variation in the residence time in R2, and the yield 40 of the protonated product 3 was determined by gas chromatogra- Yield (% 30 phy (GC).
Details
-
File Typepdf
-
Upload Time-
-
Content LanguagesEnglish
-
Upload UserAnonymous/Not logged-in
-
File Pages6 Page
-
File Size-