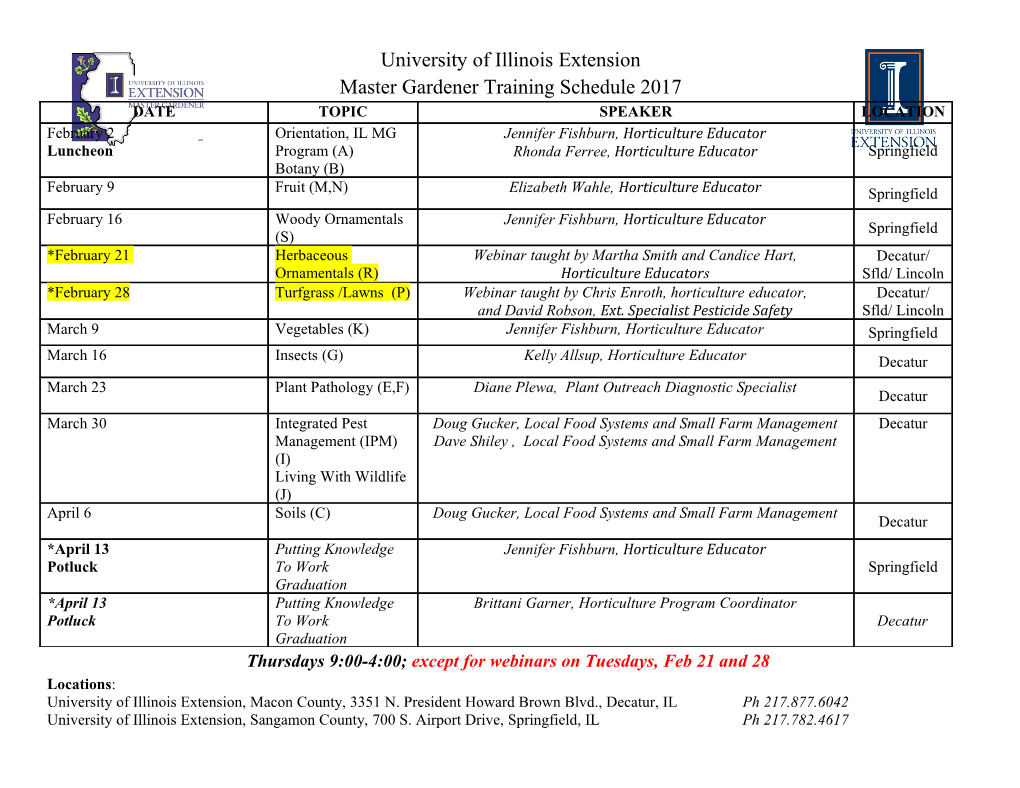
Nowland et al. BMC Genomics (2019) 20:711 https://doi.org/10.1186/s12864-019-6052-z RESEARCH ARTICLE Open Access Mitochondrial and nuclear genetic analyses of the tropical black-lip rock oyster (Saccostrea echinata) reveals population subdivision and informs sustainable aquaculture development Samantha J. Nowland1,2,4* , Catarina N. S. Silva3, Paul C. Southgate4 and Jan M. Strugnell3 Abstract Background: The black-lip rock oyster (Saccostrea echinata) has considerable potential for aquaculture throughout the tropics. Previous attempts to farm S. echinata failed due to an insufficient supply of wild spat; however, the prospect of hatchery-based aquaculture has stimulated renewed interest, and small-scale farming is underway across northern Australia and in New Caledonia. The absence of knowledge surrounding the population genetic structure of this species has raised concerns about the genetic impacts of this emerging aquaculture industry. This study is the first to examine population genetics of S. echinata and employs both mitochondrial cytochrome c oxidase subunit I gene (COI) and single nucleotide polymorphism (SNP) markers. Results: The mitochondrial COI data set included 273 sequences of 594 base pair length, which comprised 74 haplotypes. The SNP data set included 27,887 filtered SNPs for 272 oysters and of these 31 SNPs were identified as candidate adaptive loci. Data from the mitochondrial COI analyses, supports a broad tropical Indo-Pacific distribution of S. echinata, and showed high haplotype and nucleotide diversities (0.887–1.000 and 0.005–0.008, respectively). Mitochondrial COI analyses also revealed a ‘star-like’ haplotype network, and significant and negative neutrality tests (Tajima’s D=− 2.030, Fu’s Fs=− 25.638, P < 0.001) support a recent population expansion after a bottleneck. The SNP analyses showed significant levels of population subdivision and four genetic clusters were identified: (1) the Noumea (New Caledonia) sample location; (2) the Bowen (north Queensland, Australia) sample location, and remaining sample locations in the Northern Territory, Australia (n = 8) were differentiated into two genetic clusters. These occurred at either side of the Wessel Islands and were termed (3) ‘west’ and (4) ‘east’ clusters, and two migrant individuals were detected between them. The SNP data showed a significant positive correlation between genetic and geographic distance (Mantel test, P< 0.001, R2 = 0.798) and supported isolation by distance. Three candidate adaptive SNPs were identified as occurring within known genes and gene ontology was well described for the sex peptide receptor gene. Conclusions: Data supports the existence of genetically distinct populations of S. echinata, suggesting that management ofwildandfarmedstocksshouldbebaseduponmultiplemanagement units. This research has made information on population genetic structure and connectivity available for a new aquaculture species. Keywords: Population genetics, Aquaculture, Black-lip rock oyster, Genetic management, Saccostrea * Correspondence: [email protected] 1Aquaculture Unit, Department of Primary Industry and Resources, Northern Territory Government, GPO Box 3000, Darwin, NT 0801, Australia 2School of Science and Engineering, University of the Sunshine Coast, 90 Sippy Downs Drive, Sippy Downs, Queensland 4556, Australia Full list of author information is available at the end of the article © The Author(s). 2019 Open Access This article is distributed under the terms of the Creative Commons Attribution 4.0 International License (http://creativecommons.org/licenses/by/4.0/), which permits unrestricted use, distribution, and reproduction in any medium, provided you give appropriate credit to the original author(s) and the source, provide a link to the Creative Commons license, and indicate if changes were made. The Creative Commons Public Domain Dedication waiver (http://creativecommons.org/publicdomain/zero/1.0/) applies to the data made available in this article, unless otherwise stated. Nowland et al. BMC Genomics (2019) 20:711 Page 2 of 14 Background analyses of mitochondrial and SNP data revealed many For farmed marine species, understanding population subpopulations of Crassostrea virginica throughout the structure and how populations respond to the environ- Gulf of Mexico, despite its potential for high gene flow ment can inform broodstock selection, translocation [23]. Similar results of population differentiation in a boundaries, and aquaculture induced evolution [1, 2]. species with high dispersal capabilities, have been reported Potential impacts from aquaculture relate to the intro- for Crassostrea iredalei throughout Malaysia [24]and gression of exotic alleles, caused by processes such as: Ostrea edulis along the European coast [25]. Therefore, interbreeding or outbreeding of wild and cultivated without detailed population genetic analyses, predictions populations, the outcompeting of native alleles by intro- cannot be made regarding the extent of population genetic duced alleles, and translocations, all of which can nega- structure of oyster species. tively alter the genetic diversity of remnant populations The black-lip rock oyster, Saccostrea echinata (Quoy [3]. Therefore, an understanding of natural population and Gaimard, 1835), is a tropical marine bivalve that is genetics, before aquaculture occurs, is necessary for the reported to have broad geographic distribution through- proactive management of these impacts [4, 5]. Under- out the Indo-Pacific [26]. Research is currently underway standing the genetics of natural populations also pro- to improve the commercial aquaculture potential of S. vides potential benefits for improving aquaculture and echinata due to the success of small-scale farms (< 1 t biosecurity practices as many adaptive traits in the wild per annum) in northern Australia, eastern Australia, and are important production traits, such as; growth rate, New Caledonia (JR Collison pers. comm. 2018). Produc- environmental tolerances, and disease resistance [6, 7]. tion based on wild seed supply has been a bottleneck for Many methods exist to investigate population genetics, the industry [27], and this is still the case. Development and those such as mitochondrial genes provide useful of hatchery protocols for this species is therefore consid- tools for identifying and improving our understanding of ered a prerequisite for advancement and progress to relationships among species [8, 9]. More sophisticated commercial scale hatchery production is being made and increasingly affordable methods are becoming avail- [28, 29]. Larvae of S. echinata are planktonic and devel- able for application on non-model species; which often opment occurs over approximately 21 days in the hatch- lack genomic resources and includes many species used ery, after which metamorphosis and settlement takes for aquaculture [10]. The advent of next generation place [29]. Larvae may conceivably travel hundreds of sequencing technologies and restriction-site associated kilometres before settlement, which leads to the hypoth- DNA (RADseq) genotyping methods, has enabled deliv- esis that wild populations possess low levels of genetic ery of genome-wide single nucleotide polymorphisms structure [30]. However, studies on related oyster species (SNPs) for any organism (e.g. non-model species), at rea- have reported both panmictic [19, 30] and highly diver- sonable costs [11, 12]. Genome-wide SNPs can reveal gent [23, 24] population structures. To achieve the fine-scale patterns of population structure, dispersal cap- genetic conservation of oyster stocks in the face of devel- abilities, population assignment, and detect signatures of oping aquaculture efforts, a sound knowledge of popula- selection [13–15], with much higher resolution than tion genetic structure is essential. A genetic stock traditional markers (e.g. allozymes and microsatellites). assessment of natural S. echinata populations, before The use of these technologies in the detection of fine- commencement of commercial-scale aquaculture, would scale structure and signatures of selection are important provide biological information upon which responsible for determining management units, particularly in popu- management and hatchery production could be based. lations that may otherwise appear homogenous in their This study aims to fill this knowledge gap by analysing distribution [10]. both mitochondrial and nuclear DNA markers to assess Marine bivalves are characterised by large population the population genetic structure and the neutral and sizes, high fecundity, and planktonic larvae with consid- adaptive genetic diversity of S. echinata across northern erable dispersal potential [14, 16, 17]. In sessile bivalve Australia. populations, such as oysters, gene flow is achieved through the dispersal of gametes which frequently re- Results sults in high genetic diversity and weak genetic differen- Mitochondrial COI data tiation [18]. This is true for the tropical oyster The mitochondrial cytochrome c oxidase subunit I gene Crassostrea corteziensis in the Gulf of California where (COI) data set comprised 273 sequences of 594 base pair allozyme analyses revealed a single panmictic popula- (bp) length, which contained 74 different haplotypes. tion, possessing high genetic diversity [19]. However, de- Haplotype and nucleotide diversities were high (0.887– viations from this trend can occur, usually as a result of 1.000 and 0.005–0.008, respectively) and similar among larval behaviour,
Details
-
File Typepdf
-
Upload Time-
-
Content LanguagesEnglish
-
Upload UserAnonymous/Not logged-in
-
File Pages14 Page
-
File Size-