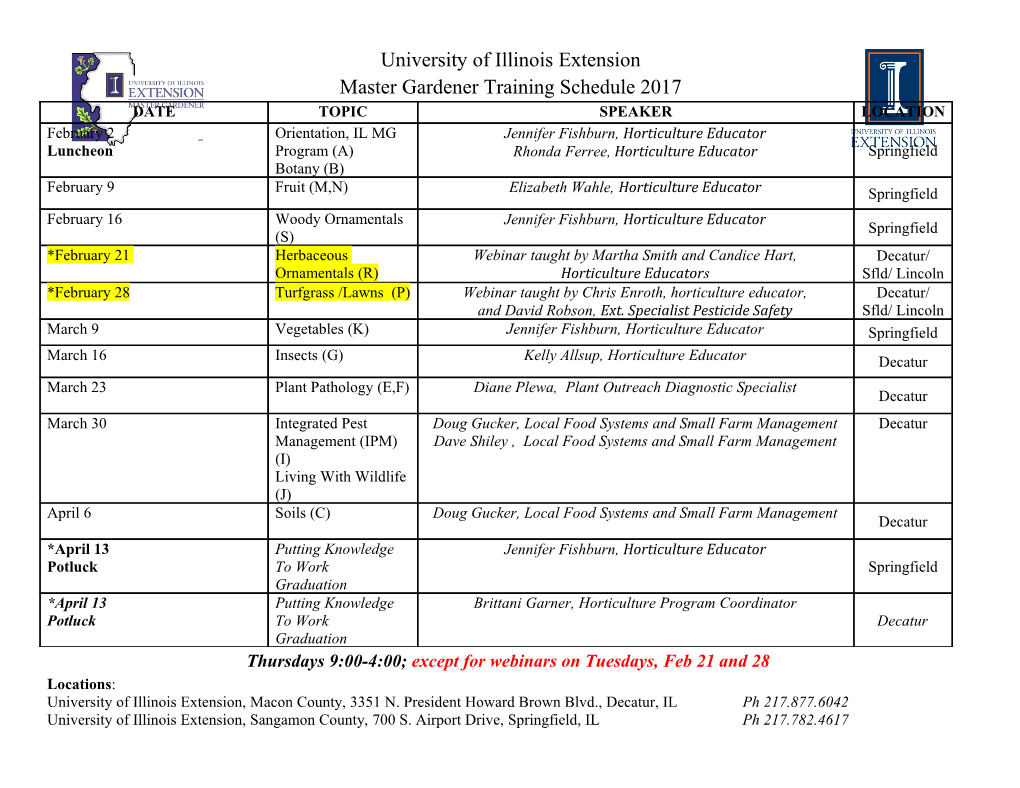
Chem. Mater. XXXX, XXX, 000–000 A DOI:10.1021/cm102144h Photoconducting Polymers for Photorefractive 3D Display Applications† Jayan Thomas,*,‡ Cory W. Christenson,‡ Pierre-Alexandre Blanche,‡ Michiharu Yamamoto,§ Robert A. Norwood,‡ and Nasser Peyghambarian‡ ‡College of Optical Sciences, The University of Arizona, Tucson, Arizona 85721, United States, and §Nitto Denko Technical Corporation, Oceanside, California 92054, United States Received July 30, 2010. Revised Manuscript Received October 23, 2010 Photorefractive composites derived from photoconducting polymers offer the advantage of dynamically recording holograms without the need for processing of any kind. Thus, they are the material of choice for many cutting edge applications, such as updatable 3D displays and imaging through a scattering medium. This article reviews the basic properties of photorefractive polymer systems and the inherent advantages that have attracted much attention. The chemistry and physics relevant for the design of the high-performance guest-host composite are discussed and recent advances emphasized. In particular, a charge transporting polymer with high mobility and history- independent response times is highlighted, as well as polymer systems useful for holographic displays and the material considerations necessary to develop high-speed, large-sensitivity composites. Introduction is also easier compared to crystals, where dopants are typically expelled during growth. The drawbacks are that Photorefractive (PR) polymers have advanced quickly they are more dispersive in nature, both optically and since their initial discovery in 1991,1 and now perform - electronically, and the mechanism behind their operation with high efficiencies and fast response times.2 4 The PR is significantly more complicated than regular crystals, effect, originally discovered in inorganic crystals more though much work has been done in these areas.9,10 than 40 years ago,5,6 initially drew attention as a perceived PR polymers now outperform inorganic counterparts detriment to nonlinear applications in these materials. How- in diffraction efficiency, two-beam coupling gain, and ever, development was pursued because of some unique sensitivity.2,11,12 properties relevant to other perceived applications. First, Because of this tremendous progress, many applica- the process was reversible though also fixable,7 allowing tions have appeared,13 including optical communica- both read/write and read-only applications, as opposed to tion,14 correlation,15 and imaging through scattering standard photographic films which could only be written - media,16 18 all with different material challenges that once. Second, the nonlocal nature of the process allowed can be met by these highly versatile polymers. Recently, coupling and energy transfer to occur between two coherent they have been shown to function in dynamic holographic beams.8 Even though there have been tremendous advance- displays,19 which are of use in medical imaging, industrial ments in the materials, these are still among the primary design, defense applications, and air traffic control, reasons photorefractives are pursued as the material of among other emerging areas such as 3D telepresence. Unlike choice for many areas. other permanent media for recording holograms, PR When the effect was discovered in organic polymers, a polymers are reversible and require no postprocessing. number of advantages over inorganics were soon realized. They demonstrate fast response time, long persistence, Organic polymer materials have the inherent advantages and high diffraction efficiency, which are necessary ma- of ready manipulation of component formulations to suit terial properties for such an application. However, prog- a given application and low cost. The structural con- ress in other areas has not been as rapid, particularly in straints were also relaxed, allowing them to be custom- the area of sensitivity. In the visible, the sensitivity is still made into different geometries, such as waveguides and orders of magnitude smaller than that of permanent films displays; significantly, samples can be made much larger used for recording static holograms. There are also few than is typical for crystals. The dielectric constant is also routes to extend operation into the infrared (IR), and only smaller, which reduces the electric field screening of recently have these begun to bear fruit. Some of these trapped charges and increases the quality factor. The include using organic photosensitizers with one photon highly customizable doping process, where specific atoms absorption in the near IR,20,21 using semiconductor nano- or molecules are added to control the electrical conductiv- crystals where the absorption band is tunable,22 and using ity, optical nonlinearities, or charge trapping properites, two-photon absorption to charge sensitizers that are 23 † Accepted as part of the “Special Issue on π-Functional Materials”. otherwise transparent. So few organic molecules under- *Corresponding author. E-mail: [email protected]. go transitions in the IR that two-photon absorption r XXXX American Chemical Society pubs.acs.org/cm B Chem. Mater., Vol. XXX, No. XX, XXXX Thomas et al. (TPA) has been used, and while more difficult experi- mentally, provides several advantages such as nonde- structive read out.24 This article reviews the basic material concepts behind fabrication of organic PR polymer composites, including the functional components, their respective roles in devi- ces, and the basic physical mechanisms that must be taken into account when designing devices. Recent progress in these areas is also discussed, including new hole- transporting polymers for reduced glass transition tempera- ture (Tg) and high mobility. Particularly, a bis-triarylamine side-chain host polymer exhibits less deep trapping lead- ing to stable dynamics independent of the illumination history. Many novel sensitizers are also reviewed, which is a very dynamic area of research. New composites with excellent sensitivity in the near IR wavelengths have extended the range of high-performing polymers beyond the visible. Finally, some material considerations neces- sary for specific applications are also taken into account, Figure 1. Schematic diagram showing the steps involved in the photo- refractive process. In the top panel, the writing beams interfere, creating such as pulsed writing for high speed operation of many an interference pattern and generating electron-hole pairs in the bright devices, and updatable holographic displays. Optimized regions. In the middle, the charges separate under the influence of an materials have been shown to exhibit good performance external electric field, which leads to a similar space-charge field and index modulation, shown together at the bottom since they are in phase. Note even under single pulse nanosecond writing times, enabling that the antinode of the modulation occurs at the node of the interference operation at 100 Hz or more, which is faster than CW because of the charge separation. recording schemes. The materials for holographic displays are discussed and extensions to reflection geometry and get trapped in the dark region. The driving force for the video-rate response times are examined, because still higher charge carriers is either diffusion due to a concentration sensitivities and trap densities are needed to accomplish gradient present in the medium or driftifanexternalelectric these goals. field is applied (generally the case for organic materials). The traps present in the material limit the migration process, Photorefractivity which can take place on micrometer length scales. Charges trapped in low intensity regions and those opposite charges In photorefractive materials, a three-dimensional re- left behind in high intensity regions give rise to an inhomo- fractive index modulation is induced by a nonuniform geneous space charge distribution. A schematic of the charge illumination. When two coherent beams intersect within generation, transport, trapping and space charge field gen- the photorefractive material, a spatially modulated in- eration is depicted in Figure 1. As given in (1), for a one- 11 tensity pattern is produced which is given by dimensional light intensity distribution, a space charge distribution F(x) in a material with a dielectric constant IðxÞ¼I0½1 þ mcosð2π=ΛÞ ð1Þ ε induces an internal space charge field Esc(x) by Poisson’s equation, which is dEsc/dx =4πF/ε. If the transport is where I0 =I1 þ I2 is the total incident intensity, i.e., the 1/2 governed by diffusion alone, the phase shift between the sum of the intensities of the two beams; m =2(I1I2) / (I þ I ) the fringe visibility and Λ the spatial wavelength or space charge field and the light intensity distribution is 1 2 π periodicity. In a tilted transmission geometry Λ is given by /2, otherwise it depends on the relative strength of the diffusion and drift processes. The final step in the photo- λ refractive grating formation is the electro-optic (EO) Λ ¼ ð Þ 2 modulation of the refractive index of the material by the 2nsin½ðR2 -R1Þ=2 internal space charge field. The phase-shift that occurs as where n is refractive index of the material, λ the optical a result of the dephasing between the initial light distribu- wavelength in vacuum, and R1 and R2 the incident internal tion and the refractive index modulation is the fingerprint angles of the two writing beams relative to the sample of photorefractivity.25 A diagram
Details
-
File Typepdf
-
Upload Time-
-
Content LanguagesEnglish
-
Upload UserAnonymous/Not logged-in
-
File Pages14 Page
-
File Size-