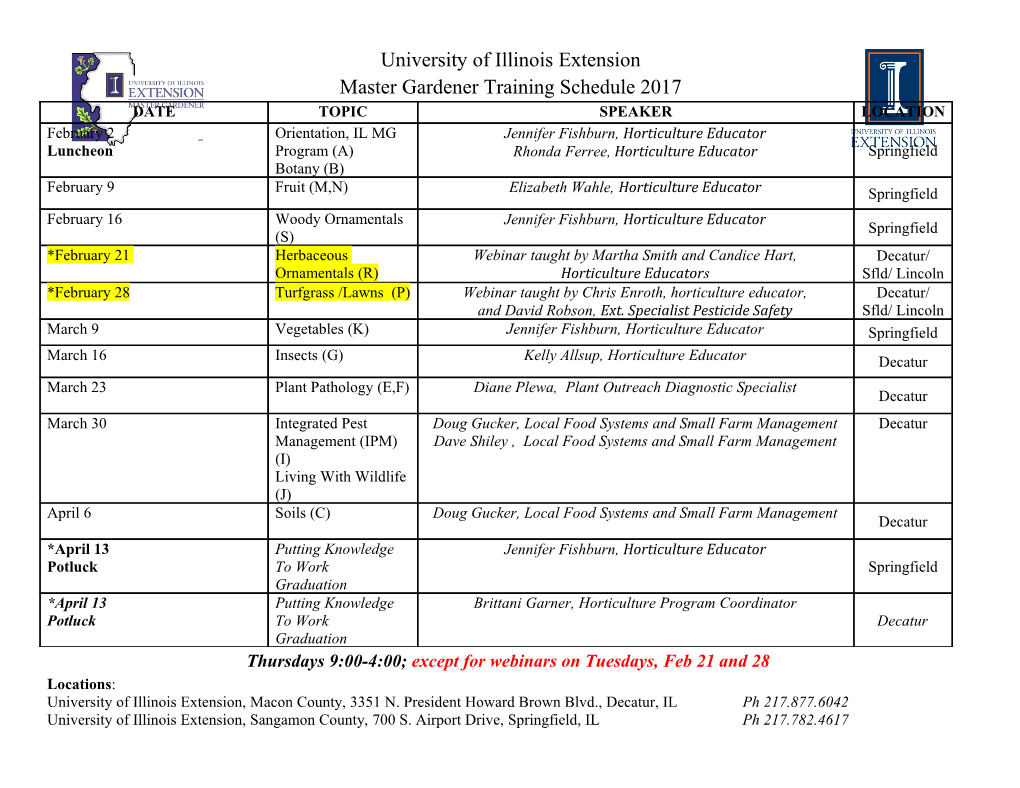
The Journal of Physical Chemistry This document is confidential and is proprietary to the American Chemical Society and its authors. Do not copy or disclose without written permission. If you have received this item in error, notify the sender and delete all copies. Cation Radicals of Hachimoji Nucleobases. Canonical Purine and Non-Canonical Pyrimidine Forms Generated in the Gas Phase and Characterized by UV-Vis Photodissociation Action Spectroscopy Journal: The Journal of Physical Chemistry Manuscript ID jp-2020-06227h.R1 Manuscript Type: Article Date Submitted by the n/a Author: Complete List of Authors: Huang, Shu; University of Washington, Chemistry Turecek, Frantisek; University of Washington, Chemistry ACS Paragon Plus Environment Page 1 of 39 The Journal of Physical Chemistry 1 2 3 Cation Radicals of Hachimoji Nucleobases. Canonical Purine and Non- 4 5 6 Canonical Pyrimidine Forms Generated in the Gas Phase and Characterized 7 8 by UV-Vis Photodissociation Action Spectroscopy 9 10 11 Shu R. Huang, František Tureček* 12 13 Department of Chemistry, Bagley Hall, Box 351700, University of Washington, Seattle, WA 14 15 98195-1700 USA 16 17 18 ABSTRACT: Oxidation of non-traditional nucleobases 1-methylcytosine (hachimoji base S) 19 20 and isoguanine (hachimoji base B) in gas-phase ternary complexes with CuII(terpyridine)2+ 21 22 formed cation radicals that were characterized by tandem mass spectrometry, UV-Vis 23 photodissociation action spectroscopy in the 210-700 nm region, and ab initio calculations up to 24 25 the CCSD(T)/complete basis set level of theory. Oxidation of S was accompanied by exothermic 26 27 isomerization in the 1-methylcytosine ion (1+●), forming 1-methylene-2-hydroxy-4- 28 +● 29 aminopyrimidine cation radical (9 ) as a non-canonical distonic isomer of the nucleobase. Ion 30 9+● was characterized by deuterium exchange experiments and provided a matching UV-Vis 31 32 action spectrum with the vibronic absorption spectrum from time-dependent density functional 33 34 theory calculations. Oxidation of B resulted in the formation of a canonical isoguanine cation 35 radical (12+●) as judged from the match of the experimental action spectrum with the calculated 36 37 vibronic absorption spectrum. The calculated adiabatic ionization energies of canonical S and B, 38 39 8.51 and 7.76 eV, respectively, indicated exothermic electron transfer from B to S+● to proceed 40 +● 41 in an ionized base pair. Contrasting this, the lowest-energy tautomer of ionized S (9 ) had a low 42 adiabatic recombination energy, RE = 5.70 eV, that would prevent it from oxidizing other 43 adiab 44 nucleobases. Recombination energies of several nucleobase tautomers are reported and 45 46 discussed. 47 48 49 1. INTRODUCTION 50 51 The information capacity of DNA, given by 4n where n is the number of coding nucleobases, has 52 53 recently been expanded by combining the four standard nucleobases, adenine (A), guanine (G) 54 cytosine (C), and thymine (T) with non-standard nucleobases isoguanine (B), 2- 55 56 57 58 1 59 60 ACS Paragon Plus Environment The Journal of Physical Chemistry Page 2 of 39 1 2 3 aminoimidazo[1,2-a]-s-triazin-4-one (5-aza-7-deazaguanine, P), 1-methylcytosine (S), 1-methyl- 4 5 2-amino-1H-pyrimidin-4-one (1-methylisocytosine), and 6-amino-5-nitro-1H-pyridin-2-one (Z).1 6 7 These nucleobases have been incorporated into the so-called hachimoji DNA and RNA as the 8 corresponding nucleotides and found to form hydrogen-bonding S:B and Z:P patterns that were 9 10 analogous to standard Watson-Crick pairing (Scheme 1).2 Considering the potential of using the 11 12 hachimoji nucleobases for expanded DNA information storage and computing, it has become of 13 14 15 16 17 18 19 20 21 22 23 24 Scheme 1. Non-traditional nucleobases S, B, Z and P for hachimoji DNA 25 26 27 28 interest to investigate their stability with respect to degradation processes resulting from 29 30 ionization. Ionization damage during long-term storage would disrupt the embedded nucleobase 31 code and result in readout malfunction and information loss. This could be particularly 32 33 pernicious, because as opposed to living organisms, DNA computers are unlikely to have 34 35 intrinsic mechanisms for damage repair. DNA damage is known to proceed via direct and 36 37 indirect mechanisms out of which direct ionization by high-energy photons or elementary 38 particles is the most prevalent one.3 Non-selective DNA ionization primarily affects the 39 40 nucleobases, forming reactive cation radicals that undergo fast electron4,5 and proton transfers6-10 41 11,12 42 among the nucleobases or by interactions with the environment. 43 In contrast to non-selective DNA ionization and side reactions occurring in the condensed 44 45 phase, the rarefied gas phase offers an inert medium where nucleobase, nucleoside, and 46 47 oligonucleotide cation radicals can be generated site-specifically and analyzed by tandem mass 48 49 spectrometry combined with ion spectroscopy methods. Besides direct ionization of gas-phase 50 nucleobases, the generation of gas-phase nucleobase cation radicals relies on intramolecular 51 52 electron transfer in doubly charged ternary transition metal complexes, such as those composed 53 54 of a nucleobase, 2:2′,6′:2″-terpyridine (terpy), and CuII. The complexes are formed by 55 56 electrospray ionization, stored in an ion trap, and the nucleobase oxidation is induced by 57 58 2 59 60 ACS Paragon Plus Environment Page 3 of 39 The Journal of Physical Chemistry 1 2 3 collisional activation which drives complex dissociation (Scheme 2). This approach to 4 5 nucleobase cation radicals has been pioneered by O'Hair and coworkers,13 following earlier 6 7 8 9 10 11 12 13 14 15 16 17 18 19 20 21 22 23 24 +● 25 Scheme 2. Formation of hachimoji cation radical S from a ternary Cu(terpy) complex. 26 27 28 studies of peptide cation radicals by Chu, Siu, and coworkers,14 and the first generation of amino 29 30 acid and peptide complexes and cation radicals in the author's laboratory.15,16 The gas-phase 31 32 cation radicals have been characterized by methods of multiphoton infrared and single-photon 33 UV-Vis photodissociation action spectroscopy. Action spectroscopy utilizes wavelength 34 35 dependent photodissociation to plot the fragment ion yield and thus construct a spectrum profile 36 37 in the wavelength domain that is representative of the active chromophore bands in the gas-phase 38 ion.17,18 By switching from absorbance measurements to monitoring photofragment ions, action 39 40 spectroscopy achieves highly sensitive detection of photon absorption even for extremely 41 42 optically thin samples, such as the 104-105 populations of ions stored in the ion trap. Previous 43 44 studies of gas-phase DNA nucleobase cation radicals revealed a diversity of structures. The 45 purine bases adenine,19 guanine,20,21 and their methyl derivatives have been shown by action 46 47 spectroscopy to form canonical tautomers of the nucleobase cation radicals, contrasting cytosine 48 49 cation radicals that were produced as a mixture of tautomers.22 Perhaps the most intriguing 50 51 result has stemmed from the generation of thymine cation radicals that have been shown by 52 action spectroscopy to predominantly assume non-canonical ion structures.23 Here, we report the 53 54 generation of nucleobase cation radicals derived from hachimoji nucleobases S and B. Using 55 56 UV-Vis action spectroscopy and ab initio theory, we wish to show that the purine nucleobase B 57 58 3 59 60 ACS Paragon Plus Environment The Journal of Physical Chemistry Page 4 of 39 1 2 3 prefers a canonical structure whereas the gas-phase pyrimidine nucleobase S is formed as a 4 5 stable non-canonical isomer. 6 7 8 2. EXPERIMENTAL SECTION 9 10 2.1. Materials and Methods. Isoguanine (Cayman Chemical, Ann Arbor, MI, USA), 1- 11 12 methylcytosine, copper nitrate, and 2:2′,6′:2″-terpyridine (Sigma-Aldrich, St. Louis, MO, USA) 13 14 were purchased from the suppliers and used as received. Complexes were made in situ from 15 equimolar concentrations of the components in 50:50 acetonitrile-water, and the solutions were 16 17 electrosprayed into a modified Bruker Daltonik (Bremen, Germany) amaZon Speed 3D ion trap 18 19 mass spectrometer. H/D exchange was accomplished in D2O/CD3CN solution and the D content 20 21 in gas-phase ions was maintained by feeding D2O solvent vapor into the dry nitrogen stream 22 flushing the enclosed electrospray interface volume. The ion trap was furnished with windows, 23 24 lenses, and mirrors to allow irradiation of trapped ions with a laser beam.24 The beam was 25 26 generated by an EKSPLA NL301G (Altos Photonics, Bozeman, MT, USA) Nd:YAG laser 27 working at 20 Hz frequency that was equipped with a PG142C optical parametric oscillator, as 28 29 described previously for a similar setup using a linear ion trap.25 The laser pulse energies were 30 31 measured at each experimental wavelength with an EnergyMax-USB J-10MB energy sensor 32 33 (Coherent Inc., Santa Clara, CA, USA) and used to calibrate the action spectra. Photofragment 34 ion intensities were measured in three sections covering the 210-700 nm range and normalized to 35 36 the number of photons per pulse. The action spectra were reproduced and are reported as 37 38 averages of two measurements performed on different days. Accurate mass measurements were 39 40 made on an Orbitrap Velos mass spectrometer (Thermo Fisher, San Jose, CA, USA) at a 41 resolving power of 60000 to assign elemental compositions to ions of interest.
Details
-
File Typepdf
-
Upload Time-
-
Content LanguagesEnglish
-
Upload UserAnonymous/Not logged-in
-
File Pages40 Page
-
File Size-