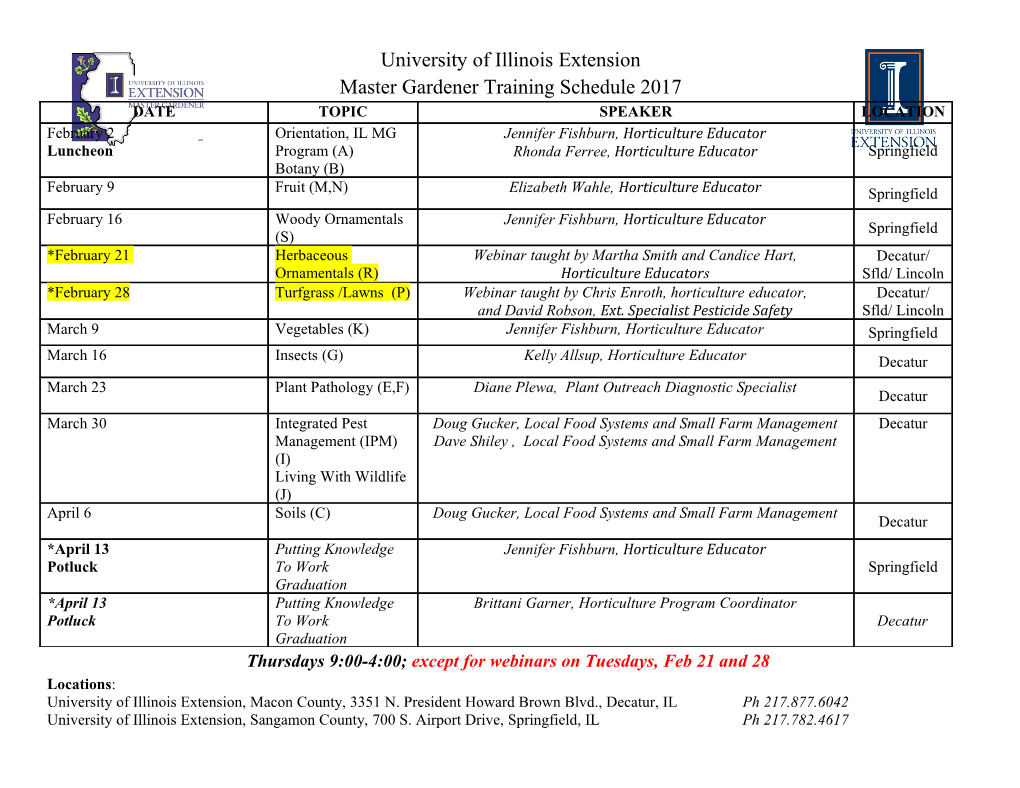
J. metamorphic Geol., 2006, 24, 451–475 doi:10.1111/j.1525-1314.2006.00648.x Characterization of polymetamorphism in the Austroalpine basement east of the Tauern Window using garnet isopleth thermobarometry F. GAIDIES,1 R. ABART,2 C. DE CAPITANI,1 R. SCHUSTER,3 J. A. D. CONNOLLY4 AND E. REUSSER4 1Department of Geosciences, University of Basel, Bernoullistrasse 30, CH-4056 Basel, Switzerland ([email protected]) 2Institute of Geological Sciences, Free University of Berlin, Malteserstrasse 74-100, D-12249 Berlin, Germany 3Geological Survey of Austria, Neulinggasse 38, A-1031 Vienna, Austria 4Earth Sciences Department, Swiss Federal Institute of Technology, CH-8092 Zurich, Switzerland ABSTRACT Garnet in metapelites from the Wo¨ lz and Rappold Complexes of the Austroalpine basement east of the Tauern Window typically shows two distinct growth zones. A first garnet generation usually forms the cores of garnet porphyroblasts and is separated by a prominent microstructural and chemical discontinuity from a second garnet generation, which forms rims of variable width. Whereas the rims were formed during the Eo-Alpine metamorphic overprint, the garnet cores represent remnants of at least two pre-Eo-Alpine metamorphic events. The pressure and temperature estimates obtained from garnet isopleth thermobarometry applied to the first growth increments of the pre-Eo-Alpine garnet cores from the Wo¨ lz and Rappold Complexes cluster into two distinct domains: (i) in the Wo¨ lz Complex, incipient growth of the first-generation garnet occurred at 4 ± 0.5 kbar and 535 ± 20 °C, (ii) in the Rappold Complex, incipient growth of the oldest garnet cores took place at 5.3 ± 0.3 kbar and 525 ± 15 °C. The Eo-Alpine garnet generation started to grow at 6.5 ± 0.5 kbar and 540 ± 10 °C. According to radiometric dating, the low-pressure garnet from the Wo¨ lz complex was formed during a Permian metamorphic event. The first-generation garnet of the Rappold Complex is probably of Variscan age. Key words: Austroalpine basement; garnet; metapelite; Rappold Complex; Wo¨ lz Complex. excess and, hence, reaction kinetics and material INTRODUCTION transport are expected to be fast. In such cases, The determination of pressure–temperature–time (P– chemical equilibrium is established rapidly and equi- T–t) paths from metamorphic mineral parageneses is librium domains may develop in the hand specimen or an important tool in unravelling the development of even on a larger scale (Carlson, 1989, 2001; Dohmen & orogenic belts (Spear, 1993). Garnet isopleth thermo- Chakraborty, 2003). However, rocks that consist of barometry has been applied to derive P–T estimates prominent compositional banding may indicate the from monometamorphic (e.g. Menard & Spear, 1993; juxtaposition of different equilibrium domains, which Vance & Mahar, 1998; Stowell et al., 2001; Zeh, 2001; has to be considered if the composition of the ther- Zeh & Holness, 2003; Evans, 2004; Kim & Bell, 2005) modynamically relevant bulk composition is to be and polymetamorphic (e.g. Zeh et al., 2004) rocks. determined. A prerequisite for the application of garnet isopleth Thermodynamic equilibrium between garnet and the thermobarometry is that thermodynamic equilibrium rock matrix may be assumed, if garnet isopleth ther- is maintained between the rim of the garnet and the mobarometry is applied to the incipient stages of gar- rock matrix during garnet growth. In this case, the net growth, i.e. if only the composition of garnet cores composition of successive garnet growth zones is solely of the oldest garnet generation present in a rock is determined by the P–T conditions as well as fluid used. In this case, equilibrium-phase relations that are composition for any given thermodynamically relevant calculated by free-energy minimization for a system bulk composition. The latter is defined as the volume with specified bulk composition (e.g. de Capitani & domain in a rock, over which equilibrium is main- Brown, 1987; Powell & Holland, 1993; Connolly & tained during garnet growth. Garnet growth in previ- Petrini, 2002) and an internally consistent thermo- ously unmetamorphosed hydrous rocks or in rocks dynamic database (e.g. Berman, 1988; Gottschalk, that have only experienced low-grade metamorphism 1997; Holland & Powell, 1998) can be used as theore- generally occurs by dehydration reactions. During tical predictions of the equilibrium assemblages that dehydration, an aqueous fluid is likely to be present in would develop if the corresponding rock maintained Ó 2006 Blackwell Publishing Ltd 451 452 F. GAIDIES ET AL. equilibrium and the bulk composition is appropriate. Table 1. Sample locations and GPS coordinates. The P–T conditions that prevailed during the initial a stages of garnet growth can then be derived from Coordinates comparison of the observed garnet compositions and Sample ENAltitude (m) Unit the equilibrium assemblage diagrams, including con- 10F03 014°13¢15¢¢ 47°19¢16¢¢ 2030 Wo¨ lz tours for garnet composition, calculated for the 11F03 014°13¢20¢¢ 47°19¢20¢¢ 2060 Wo¨ lz appropriate bulk-rock composition. 12F03 014°13¢20¢¢ 47°19¢20¢¢ 2060 Wo¨ lz 16F03 014°13¢00¢¢ 47°19¢08¢¢ 1950 Wo¨ lz A second prerequisite for the application of garnet 28F03 014°15¢44¢¢ 47°18¢39¢¢ 2010 Wo¨ lz isopleth thermobarometry is that the original growth 29F03A + B 014°15¢48¢¢ 47°18¢49¢¢ 2020 Wo¨ lz zoning of garnet is preserved in its original state, i.e. 32F03 014°15¢52¢¢ 47°19¢03¢¢ 2230 Wo¨ lz 37F03 014°24¢28¢¢ 47°23¢17¢¢ 2060 Wo¨ lz that modification of the original chemical zoning pat- 39F03 014°24¢11¢¢ 47°23¢24¢¢ 2040 Wo¨ lz tern by intracrystalline diffusion is negligible. This is a 40F03-1 014°24¢11¢¢ 47°23¢25¢¢ 2060 Wo¨ lz 40F03-2 014°24¢11¢¢ 47°23¢25¢¢ 2060 Wo¨ lz reasonable assumption as long as amphibolite facies 41F03 014°24¢03¢¢ 47°23¢29¢¢ 2090 Wo¨ lz conditions are not surpassed, because of the exceed- 42F03 014°24¢02¢¢ 47°23¢29¢¢ 2100 Wo¨ lz ingly slow rates of cation diffusion in garnet (Chakra- 43F03 014°24¢02¢¢ 47°23¢29¢¢ 2100 Wo¨ lz 44F03 014°23¢54¢¢ 47°23¢33¢¢ 2130 Wo¨ lz borty & Ganguly, 1992). Hence, for a persuasive 19F03 014°12¢46¢¢ 47°18¢53¢¢ 1790 Rappold modification of the original chemical pattern at low to 24F03 014°12¢46¢¢ 47°18¢42¢¢ 1825 Rappold moderate temperatures to occur, garnet has to be ex- 31F03 014°15¢49¢¢ 47°19¢00¢¢ 2170 Rappold 33F03 014°15¢52¢¢ 47°19¢06¢¢ 2265 Rappold posed to these temperatures for extraordinary long 35F03 014°15¢46¢¢ 47°19¢15¢¢ 2170 Rappold durations. In contrast, only the short-term exposure of garnet to temperatures exceeding amphibolite facies aWorld Geodetic System 84 (WGS84). conditions prevents them from effective modification of the original zoning pattern. There is general agreement erations preserved, employing bulk-rock chemistries on the presumption that the chemical zoning patterns and garnet as the only relic of the preexisting mineral of garnet porphyroblasts provide reliable records of the parageneses. Furthermore, it will be shown that the P–T evolution up to upper amphibolite facies condi- integration of garnet isopleth thermobarometry with tions (e.g. Ayres & Vance, 1997). However, Stowell the Rayleigh fractionation model of Evans (2004) en- et al. (1996) demonstrated that in addition to intra- ables detailed P–T paths to be predicted for garnet crystalline diffusion other processes such as Ca-me- growth in the course of different orogenic cycles. The tasomatism might affect the original growth zoning of results obtained help to discriminate between distinc- garnet. tive metamorphic events and, hence, provide insights As a result of the considerably slow intracrystalline into the polymetamorphic evolution in the Eastern diffusion in garnet at low to moderate temperatures, Alps. the components which are incorporated into garnet during growth, are effectively removed from the rock matrix (e.g. Vance & Mahar, 1998; Evans, 2004). In GEOLOGICAL SETTING general, certain components such as Mn or Fe are The Eastern Alps form a 500-km-long east–west- preferentially fractionated into garnet. This leads to a trending fold and thrust belt. They developed in the successive depletion of these components in the rock course of two distinct continent–continent collision matrix and may change the effective bulk-rock com- events during the Cretaceous and Tertiary (Froitzheim position during garnet growth. This effect is partic- et al., 1996). According to Schmid et al. (2004), three ularly pronounced if the modal amount of newly major plate-tectonic units can be distinguished in the formed garnet is high. The fractionation effect must be Eastern Alps: the Helvetic realm and the sub-Penninic accounted for, if garnet isopleth thermobarometry is nappes are considered to represent one major unit used to reconstruct P–T paths from the compositions which corresponds to the former southern European of successive garnet growth increments. The progres- margin. The Penninic nappes represent the Jurassic sive modification of the thermodynamically relevant and Cretaceous oceanic crust and micro-continents, bulk-rock composition during garnet growth may be and the Austroalpine nappes represent the frontal part estimated from a Rayleigh fractionation model (Hol- of the Apulian continental microplate. In the Creta- lister, 1966; Atherton, 1968; Evans, 2004). ceous, the Apulian microplate experienced internal In this study, we present an application of garnet deformation because of a WNW–ESE-directed intra- isopleth thermobarometry to polymetamorphic garnet continental collision event, which is referred to as the porphyroblasts of metapelites from the Wo¨ lz and Eo-Alpine event. Cretaceous tectonics led to the for- Rappold Complexes of the Austroalpine crystalline mation of large de´collement nappes in the uppermost basement east of the Tauern Window (Niedere Tauern, crustal levels and substantial shortening and subduc- Eastern Alps) (Table 1). It will be demonstrated that tion in the deeper basement units.
Details
-
File Typepdf
-
Upload Time-
-
Content LanguagesEnglish
-
Upload UserAnonymous/Not logged-in
-
File Pages25 Page
-
File Size-