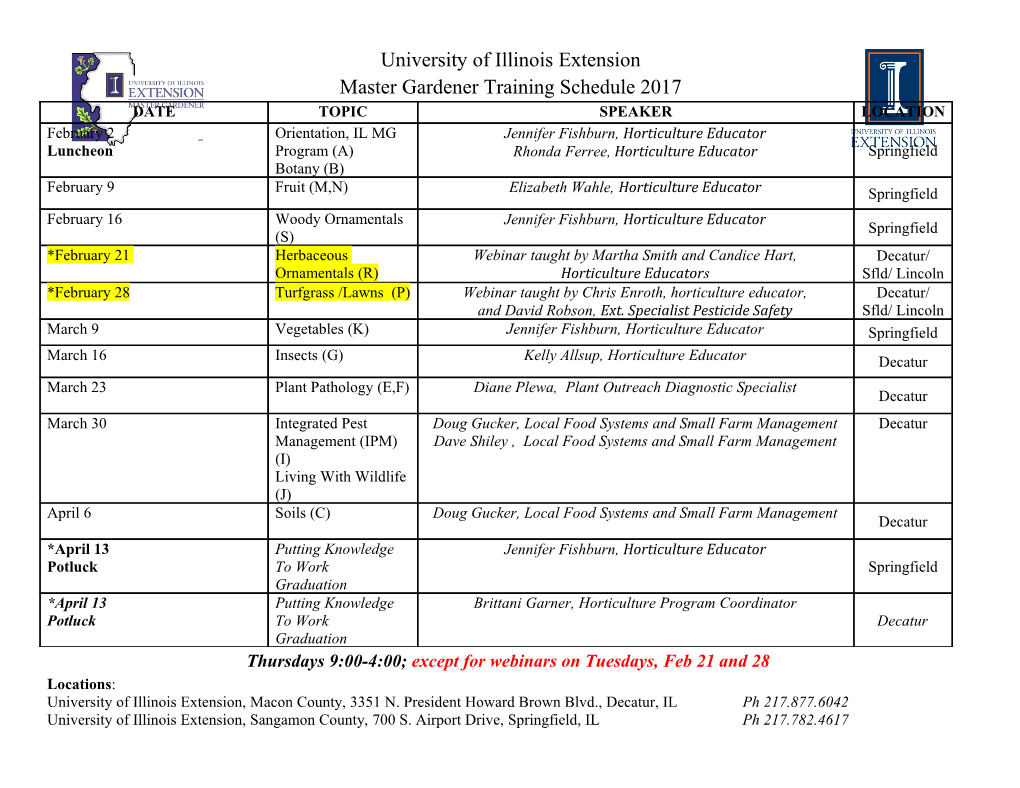
6.3. Micro and Mesoporous Materials According to the classification made by IUPAC1, porous solids can be grouped into three categories, depending on their pore diameter: microporous (d < 2 nm), mesoporous (2 nm < d < 50 nm), and macroporous (d > 50 nm) materials. Almost all of zeolites and their derivatives are microporous, whereas surfactant templated mesoporous materials and most xerogels and aerogels are mesoporous materials. In this section, we will briefly introduce these meso and microporous materials and their respective synthesis techniques. This field has been extensively covered with excellent reviewed articles2,3. 6.3.1. Ordered Mesoporous Structures Ordered mesoporous materials are made with a combination of using self-assembled surfactants as template and simultaneous sol-gel condensation around template. Mesoporous materials may have many important technological applications as supports, adsorbents, sieves, or nanoscale chemical reactors. Such materials have uniformly sized and shaped pores with diameters ranging from 3 nm to several tens nanometers and microns long, and often have a very large pore volume (up to 70%) and very high surface area (> 700 m2/g). Before we discuss the details of the synthesis of ordered mesoporous materials, a brief introduction to surfactants and the formation of micelles. Surfactants are organic molecules, which comprise two parts with different polarity4. One part is a hydrocarbon chain (often referred to as polymer tail), which is nonpolar and hence hydrophobic and lipophilic, whereas the other is polar and hydrophilic (often called hydrophilic head). Because of such a molecular structure, surfactants tend to enrich at the surface of a solution or interface between aqueous and hydrocarbon solvents, so that the hydrophilic head can turn towards the aqueous solution, resulting in a reduction of surface or interface energy. Such concentration segregation is spontaneous and thermodynamically favorable. Surfactant molecules can be generally classified into four families, and they are known as anionic, cationic, nonionic, and amphoteric surfactants, which are briefly discussed below: 1. Typical anionic surfactants are sulfonated compound with a general formula R-SO3Na, and sulfated compounds of R-OSO3Na, with R being an alkyl chain consisting of 11 to 21 carbon atoms. 2. Cationic surfactants commonly comprise of an alkyl hydrophobic tail and a methyl- ammonium ionic compound head, such as cetyl trimethyl ammonium bromide (CTAB), C16H33N(CH3)3Br and cetyl trimethyl ammonium chloride (CTAC), C16H33N(CH3)3Cl. 3. Nonionic surfactants do not dissociate into ions when dissolved in a solvent as both anionic and cationic surfactant. Their hydrophilic head is a polar group such as ether, R- O-R, alcohol, R-OH, carbonyl, R-CO-R, and amine, R-NH-R. 4. Amphoteric surfactants have properties similar to either nonionic surfactants or ionic surfactants. Examples are betaines and phospholipides. 1 When surfactants dissolve into a solvent forming a solution, the surface energy of the solution will decrease rapidly and linearly with an increasing concentration. This decrease is due to the preferential enrichment and the ordered arrangement of surface of surfactant molecules on the solution surface i.e., hydrophilic heads inside the aqueous solution and/or away from nonpolar solution or air. However, such a decrease stops when a critical concentration is reached, and the surface energy remains constant with further increase in the surfactant concentration, as shown in Figure 6.7. This figure also shows that surface energy of a solution changes with the addition of general organic or inorganic solutes. The critical concentration in Figure 6.7 is termed as the critical micellar concentration, or CMC. Below the CMC, the surface energy decreases due to an increased coverage of surfactant molecules on the surface as the concentration increases. At the CMC, the surface has been fully covered with the surfactant molecules. Above the CMC, further addition of surfactant molecules leads to phase segregation and formation of colloidal aggregates, or micelles5. The initial micelles are spherical and individually dispersed in the solution, and would transfer to a cylindrical rod shape with further increased surfactant concentration. Continued increase of surfactant concentration results in an ordered parallel hexagonal packing of cylindrical micelles. At a still higher concentration, lamellar micelles would form. Inverse micelles would form at an even higher concentration. Figure 6.8 are schematics of various micelles formed at various surfactant concentrations above the CMC. Micelles, particularly hexagonal or cubic packing of cylindrical micelles have been used as templates to synthesize ordered mesoporous materials through sol-gel processing6. The formation of this new family of materials was first reported in 19927,8. The first ordered mesoporous materials synthesized were denoted as MCM-41 and MCM-48. MCM-41 is an aluminosilicate possessing hexagonally arranged one-dimensional pores with diameters ranging from 1.5 to 10 nm, and MCM-48 is an aluminosilicate with a three-dimensional pore system and diameters of order of 3 nm. It should be noted that the inorganic portion of mesoporous materials MCM-41 and MCM-48 are amorphous aluminosilicates. The process is conceptually straightforward and can be briefly described below. Surfactants with a certain molecule length are dissolved into a polar solvent with a concentration exceeding its CMC, mostly at a concentration, at which hexagonal or cubic packing of cylindrical micelles is formed. At the same time, the precursors for the formation of desired oxide(s) are also dissolved into the same solvent, together with other necessary chemicals such as catalyst. Inside the solution, several processes proceed simultaneously. Surfactants segregate and form micelles, whereas oxide precursors undergo hydrolysis and condensation around the micelles simultaneously, as schematically shown in Figure 6.9. Various organic molecules including surfactants and block copolymers have been used to direct the formation of ordered mesoporous materials9,10,11,12. Various oxides other than silica and aluminosilicates are found to form ordered mesoporous structures13,14,15,16,17,18,19. A lot of research has been conducted in the synthesis of ordered mesoporous complex metal oxides13,20,21, also called mixed metal oxides, which possess many important physical properties conducive to a wide range of applications, particularly as heterogeneous catalysts in modern chemical industry. The greatest challenge in the synthesis of ordered mesoporous complex metal oxides is the same as the formation of nanoparticles and nanowires of complex metal oxides by sol-gel processing, which is to ensure the formation of homogeneous desired stoichiometric composition 2 through hetero-condensation. All the general considerations that have been discussed previously are applicable here. However, the situation here is even more complex, since the presence of surfactants in the solution would complicate the reaction kinetics of hydrolysis and condensation reactions. Some surfactants would act as catalysts to promote hydrolysis and condensation reactions. The presence of relatively large surfactant molecules and micelles in the solution would certainly have a steric effect on the diffusion process. Although all these surfactant effects are present in the synthesis of single metal oxide mesoporous materials, a given surfactant may have varied degree effects on different precursors. Therefore, the influences of surfactants on the hydrolysis and condensation reactions in the formation of ordered mesoporous complex metal oxides should be carefully considered. Table 6.1 summarizes some physical properties of mesoporous complex oxides and Figure 6.10 shows TEM images of various mesoporous materials. Optically transparent and electronic conductive complex oxide, indium tin oxide (ITO), has also been studied to form mesoporous structure22. In the fabrication of mesoporous ITO, A prime impediment is used to control the competing hydrolysis and condensation reactions, which is achieved by employing atrane complexes as precursors to slow the kinetics of hydrolysis. Indium acetate and tin isopropoxide with desired stiochiometric ratios was dissolved in a 10-fold molar excess of triethanolamine under an inert nitrogen atmosphere. Approximately 10 vol% dry formamide was also added to lower the viscosity. After the solution was mixed for 4 h, CTAB in a 3.5:1 molar ratio with respect to the total metal concentration was admixed to the solution, and the pH was adjusted to 8 with 4 M sodium hydroxide. The mixture was held at 80 °C for 96 h prior to filtering off the product. The resultant ITO powder with a In:Sn molar ratio of 1:1 has a BET surface area of 273 m2/g and a pore diameter of ~ 2 nm as determined by nitrogen sorption isotherms. XRD indicates the formation of crystalline ITO after calcinations at unspecified temperatures, and TEM image shows a worm-hole topography. However, electrical conductivity measurements taken on a water-free pressed pellet showed an average value of σ = 1.2 x 10-3 S/cm at room temperature, which is about 3 orders of magnitude lower than that of ITO thin films under the same condition. Table 6.1. Physical properties of mesoporous complex metal oxides Oxide Pore size (nm) BET surface BET surface Porosity (%) area (m2/g) area (m2/cm3) SiAlO3.5 6 310 986 59 Si2AlO5.5 10 330 965 55 SiTiO4 5 495 1638 63
Details
-
File Typepdf
-
Upload Time-
-
Content LanguagesEnglish
-
Upload UserAnonymous/Not logged-in
-
File Pages25 Page
-
File Size-