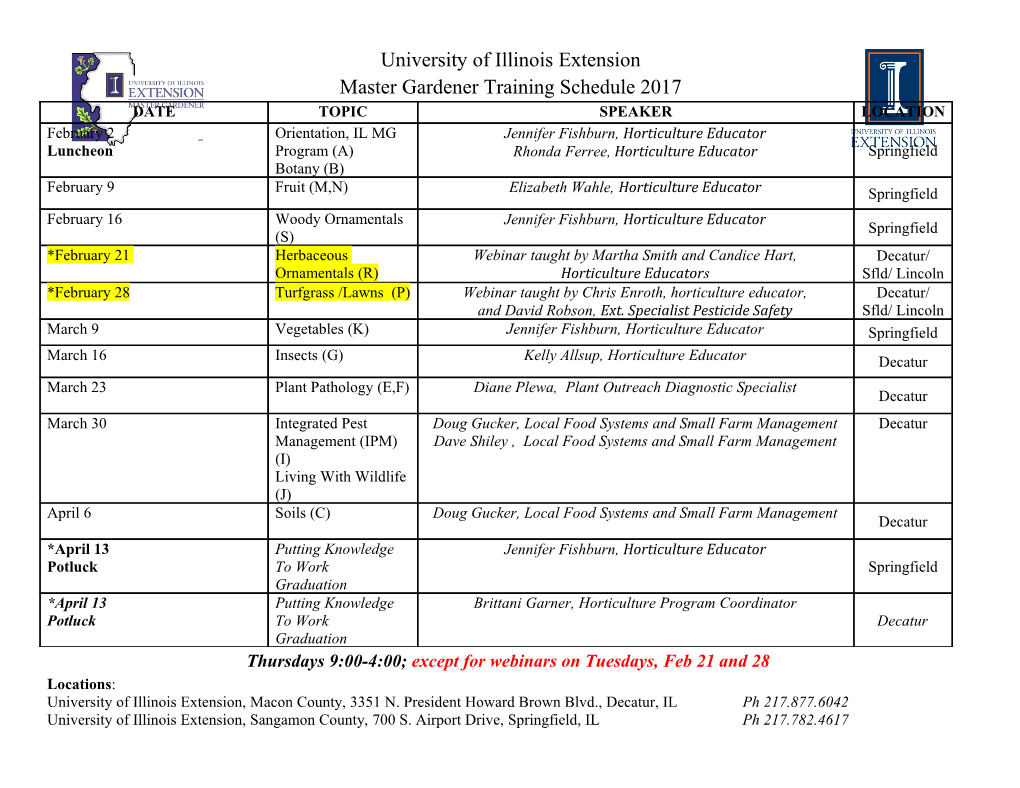
Atmos. Chem. Phys., 12, 6237–6271, 2012 www.atmos-chem-phys.net/12/6237/2012/ Atmospheric doi:10.5194/acp-12-6237-2012 Chemistry © Author(s) 2012. CC Attribution 3.0 License. and Physics Halogen activation via interactions with environmental ice and snow in the polar lower troposphere and other regions J. P. D. Abbatt1, J. L. Thomas2,3, K. Abrahamsson4, C. Boxe5, A. Granfors4, A. E. Jones6, M. D. King7, A. Saiz-Lopez8, P. B. Shepson9, J. Sodeau10, D. W. Toohey11, C. Toubin12, R. von Glasow13, S. N. Wren1, and X. Yang14,15 1Department of Chemistry, University of Toronto, Toronto, ON, M5S 3H6, Canada 2UPMC Univ. Paris 06, Universite´ Versailles St-Quentin, CNRS/INSU, UMR8190, LATMOS-IPSL, Paris, France 3University of California, Los Angeles, Department of Atmospheric and Oceanic Sciences, Los Angeles, CA 90095, USA 4Department of Chemistry and Molecular Biology, University of Gothenburg, 412 96 Gothenburg, Sweden 5Department of Physical, Environmental and Computer Science, Medgar Evers College-City University of New York, 1650 Bedford Avenue, Brooklyn, NY 11235, USA 6British Antarctic Survey, Natural Environment Research Council, High Cross, Madingley Road, Cambridge, CB3 0ET, UK 7Department of Earth Sciences, Royal Holloway, University of London, Egham, Surrey, TW20 0EX, UK 8Laboratory for Atmospheric and Climate Science, CSIC, Toledo, Spain 9Dept. of Chemistry and Earth and Atmospheric Sciences, Purdue University, West Lafayette, IN, USA 10Department of Chemistry and Environmental Reserach Institute, University College Cork, Cork, Ireland 11Department of Atmospheric and Oceanic Sciences, University of Colorado, Boulder, CO, USA 12Laboratoire de Physique des Lasers, Atomes et Molecules, CNRS, UMR8523, Centre d’Etudes et de Recherches Lasers et Applications (CERLA), Universite des Sciences et Technologies de Lille, 59655 Villeneuve d’Ascq Cedex, France 13School of Environmental Sciences, University of East Anglia, Norwich, UK 14Centre for Atmospheric Science, Department of Chemistry, University of Cambridge, Cambridge, CB2 1EW, UK 15National Centre for Atmospheric Science (NCAS)-Climate, UK Correspondence to: J. P. D. Abbatt ([email protected]) and J. L. Thomas ([email protected]) Received: 15 March 2012 – Published in Atmos. Chem. Phys. Discuss.: 5 April 2012 Revised: 27 June 2012 – Accepted: 28 June 2012 – Published: 19 July 2012 Abstract. The role of ice in the formation of chemically ac- is highlighted in the presentation of work from the molecular tive halogens in the environment requires a full understand- to the regional scale, with a focus on understanding funda- ing because of its role in atmospheric chemistry, including mental processes. This is essential for developing realistic controlling the regional atmospheric oxidizing capacity in parameterizations and descriptions of these processes for in- specific situations. In particular, ice and snow are important clusion in larger scale models that are used to determine their for facilitating multiphase oxidative chemistry and as media regional and global impacts. upon which marine algae live. This paper reviews the na- ture of environmental ice substrates that participate in halo- gen chemistry, describes the reactions that occur on such sub- strates, presents the field evidence for ice-mediated halogen 1 Introduction activation, summarizes our best understanding of ice-halogen activation mechanisms, and describes the current state of Ice and snow substrates, while long considered to be by- modeling these processes at different scales. Given the rapid standers in environmental chemistry, are now known to play pace of developments in the field, this paper largely addresses important roles in a variety of atmospheric chemical phe- advances made in the past five years, with emphasis given to nomena ranging from the scavenging of volatile organic the polar boundary layer. The integrative nature of this field compounds by falling snow (Lei and Wania, 2004), to partici- pation in photoreduction processes associated with deposited Published by Copernicus Publications on behalf of the European Geosciences Union. 6238 J. P. D. Abbatt et al.: Halogen activation via interactions with environmental ice and snow mercury (Durnford and Dastoor, 2011), and to sequestration addition, due to changes in HOx cycling, OH levels them- of persistent organic pollutants (Brown and Wania, 2008). selves may be affected (e.g. Sjostedt et al., 2007). Recently, This review focuses on the role that ice and snow play in it has been shown that a large degree of variability in ozone the formation of volatile halogen-containing molecules in the in the Arctic is correlated with exposure to sea ice (Gilman coldest parts of our atmosphere, for example in the bound- et al., 2010; Jacobi et al., 2010), which likely indicates cat- ary layer at high latitudes and the upper troposphere-lower alytic loss of ozone by halogens. stratosphere (UT-LS). It is likely that similar processes occur Ozone levels in the UT-LS can also be affected by het- in all snow-covered regions of the globe. erogeneous reactions such as Reactions (R1) and (R2) occur- Ice-halogen chemistry was first explored for its impor- ring on ice and other particle surfaces (Borrmann et al., 1996, tance to the Antarctic ozone hole, a phenomenon in which 1997). Very low temperatures in the UT-LS promote forma- the majority of ozone within the polar stratospheric vortex tion of thin cirrus clouds over a large fraction of the Earth’s is depleted annually for months at a time (summarized in surface area. Very short-lived substances (VSLS) containing Solomon, 1999). A conceptual advance in our understanding halogens decompose to release halogen atoms in this region. of atmospheric chemistry arose through coupled laboratory, However, air masses in the UT-LS are not as dynamically iso- field and modeling studies that demonstrated that interactions lated as they are in the polar stratosphere and boundary layer with polar stratospheric clouds convert relatively long-lived in winter and spring, so attribution of ozone depletion to ice halogenated species to more reactive species that ultimately chemistry in the UT-LS is difficult. lead to ozone destruction. For example, compounds such as While the gas phase reactions for destruction of boundary ClONO2, HOCl and HCl adsorb to cloud surfaces, and are layer ozone by halogen radicals are analogous to those that converted via heterogenous reactions to gas phase Cl2 which proceed in the polar stratosphere, the sources of the halogens is readily photolyzed: to the gas phase and the means by which they can be recy- cled and maintained in reactive forms are distinctly differ- ClONO2 + HCl → HNO3 + Cl2 (R1) ent. In the stratosphere, where residence times can be years and where photodissociation rates are enhanced by the en- HOCl + HCl → Cl2 + H2O (R2) ergetic UV fields, the halogen sources are well known and include both chlorofluorocarbons and halons. By contrast, in This chemistry largely occurs on particles that are not com- the polar boundary layer where photodissociation of small posed of pure ice, but rather solid nitric acid hydrates or su- brominated organics of biological origin does not proceed percooled solutions of sulfuric and nitric acid. This multi- fast enough at low solar angles, the focus of most recent re- phase chemistry has similarities to that which prevails in the search has been on chemistry occurring on saline surfaces polar boundary layer on sea ice surfaces, blowing snow and including both the snowpack and sea ice surfaces. Reactive the snowpack, as well as potentially in the UT-LS on ice crys- processes, either driven photochemically or by gas-surface tals. In particular, the chemistry in Reaction (R2) proceeds chemical interactions, have been studied as a means by which on surfaces and in the bulk, likely through ionic processes sea salt halides are oxidized to more reactive forms. For facilitated by the dissociation of HCl on the ice surface, as example, one of a number of processes identified involves described below. HOBr oxidation of bromide, which can either recycle active Research related to ice chemistry in the Arctic and Antarc- bromine or lead to its autocatalytic release (Fan and Jacob, tic boundary layers has accelerated in recent decades, mo- 1992; Mozurkewich, 1995; Vogt et al., 1996): tivated by observations of ozone depletion events (ODEs) HOBr + HBr/Br− → Br + H O/OH− (R3) that coincide with elevated levels of atmospheric brominated 2 2 compounds (Barrie et al., 1988), now known to be largely This is a multiphase process which, depending upon the sub- reactive species such as BrO, Br and HOBr (as summarized strate, may or may not proceed faster under acidic conditions. in Simpson et al., 2007b). Ozone loss can occur rapidly via In particular, as described later in the paper, there is evidence well-known gas phase catalytic processes involving bromine that some bulk reactions require acidity to proceed whereas radicals, such as Br and BrO. In addition, measurements of a number of interfacial ones do not. active iodine in polar regions have been made (e.g. Saiz- The role of bromine in boundary layer ODEs was recog- Lopez et al., 2007b). nized very early on, and it motivated early research in the Formation of reactive halogens and subsequent destruction field. More recently, however, the complexity of the interre- of ozone significantly changes the oxidizing conditions of lationships between gas-surface chemistry, photochemistry, the boundary layer. For example, mercury compounds, oxi- and biological processes has been recognized (Domine´ and dized by halogen radicals, are deposited to the surface during Shepson, 2002). For example, it is now known that nitrate in ODEs (Schroeder et al., 1998; Steffen et al., 2008). Simi- snow photodissociates into reactive nitrogen forms (Honrath larly, reactions with halogen radicals reduce the lifetimes of et al., 2002; Beine et al., 2008), a process that generates OH dimethyl sulphide and other organic compounds that are nor- radicals within the snowpack. Similarly, H2O2 photolysis is mally controlled by OH (von Glasow and Crutzen, 2004).
Details
-
File Typepdf
-
Upload Time-
-
Content LanguagesEnglish
-
Upload UserAnonymous/Not logged-in
-
File Pages35 Page
-
File Size-