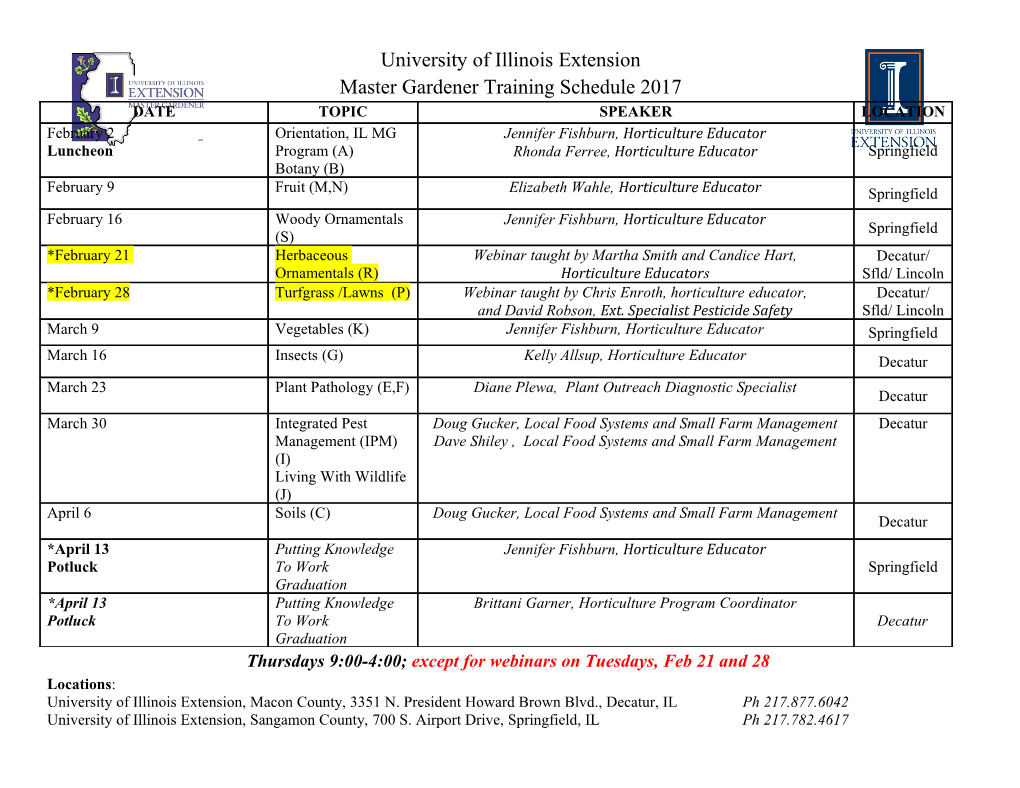
Hydrobiologia DOI 10.1007/s10750-016-3005-2 ROTIFERA XIV Vulnerability of rotifers and copepod nauplii to predation by Cyclops kolensis (Crustacea, Copepoda) under varying temperatures in Lake Baikal, Siberia Michael F. Meyer . Stephanie E. Hampton . Tedy Ozersky . Olga O. Rusanovskaya . Kara H. Woo Received: 22 March 2016 / Revised: 24 September 2016 / Accepted: 1 October 2016 Ó Springer International Publishing Switzerland 2016 Abstract As lakes warm worldwide, temperature that the less evasive Gastropus and Keratella would be may alter plankton community structure and abun- more susceptible to predation than nauplii. We dance by affecting not only metabolism but also exposed a starved predator to individuals of each prey trophic interactions. Siberia’s Lake Baikal presents type and observed encounters, ingestions, and escapes. special opportunity for studying shifting trophic Contrary to our hypothesis, Keratella were consumed interactions among cryophilic zooplankton species in at lower rates than nauplii, due to higher probability of a rapidly warming lake. To understand how warming ingestion after encounter with nauplii. In a second may affect trophic interactions among plankton, we experiment, we assessed how predation varied across a studied predator–prey relationships of a copepod thermal gradient, confining all three prey types and predator (Cyclops kolensis) with three prey types: one starved predator at 5° temperature increments two rotifer species (Gastropus stylifer and Keratella (5–20°C). Predation outcomes mirrored observational cochlearis) and copepod nauplii. We hypothesized feeding trials, and predation outcomes were indepen- dent of temperature. Rotifers’ relatively high repro- ductive rate may present a mechanism to withstand Guest editors: M. Devetter, D. Fontaneto, C. D. Jersabek, predation should copepod’s preferred nauplii prey D. B. Mark Welch, L. May & E. J. Walsh / Evolving rotifers, become less abundant in a warmer Baikal. evolving science M. F. Meyer (&) Keywords Freshwater food webs Á Rotifera Á School of the Environment, Washington State University, Coldwater stenotherms Á Zooplankton Pullman, WA, USA e-mail: [email protected] S. E. Hampton Á K. H. Woo Introduction Center for Environmental Research, Education, and Outreach, Washington State University, Pullman, WA, As lakes warm worldwide (O’Reilly et al., 2015), USA aquatic communities are likely to shift in composition T. Ozersky and interactions (Moore et al., 1996), both as a result Large Lakes Observatory, University of Minnesota- of direct effects on metabolism (Huey, 1991; Dell Duluth, Duluth, MN, USA et al., 2011) and indirect effects mediated through trophic pathways (Dell et al., 2014). The variety of O. O. Rusanovskaya Biological Research Institute, Irkutsk State University, responses to warming magnifies potential for relation- Irkutsk, Russian Federation ships, such as competition and predation, to change 123 Hydrobiologia under warmer or more variable temperature regimes with warm-adapted, cosmopolitan species (Kozhov, (Huey, 1991; Seifert et al., 2015), but these changes 1963; Kozhova & Izmest’eva, 1998; Hampton et al., may be difficult to predict. Temperature-induced 2008, 2014; Izmest’eva et al., 2016). Warming in changes in planktonic composition and activity have Siberia has occurred nearly twice as fast as global air important implications for ecosystem functioning temperatures, and Lake Baikal has warmed approxi- (e.g., Elliott et al., 2006), determining carbon flow to mately twice as fast as ambient air temperature, with higher trophic levels (Schabetsberger et al., 2009; average surface temperatures increasing by 0.2°C Schmidt et al., 2009), nutrient cycling (Lehman, 1980; decade-1 since 1946 (Shimaraev et al., 2002; Hamp- Higgins et al., 2006; Hambright et al., 2007), occur- ton et al., 2008). Furthermore, increases in cosmopoli- rence of nuisance algal blooms (Rigosi et al., 2015), tan plankton abundances during summer have been and greenhouse gas production (Tadonle´ke´ et al., observed, such as the 12-fold increase of Cyclops 2012). Furthermore, temperature-mediated changes kolensis Lilljeborg, 1901 over the past six decades can alter plankton assemblage composition and abun- (Izmest’eva et al., 2016). Concurrent with this warm- dance, as well as interactions among planktonic ing, endemic plankton species abundance has either species, which can eventually contribute to temporal not changed or has declined in summer months mismatching of consumers with their resources (Hampton et al., 2008; Izmest’eva et al., 2016). The (Thackeray et al., 2010). These effects of temperature extent, to which these changes may have occurred change on communities can become even more through direct effects on metabolism, or indirectly complex when metabolic rate and overall activity of through food web changes, is unknown. aquatic organisms differ across taxa (Dell et al., 2014). Here we experimentally explored the potential for For example, a rotifer trophic web comprised of trophic interactions between rotifers and their preda- predatory Asplanchna brightwellii Gosse, 1850, her- tors to change with shifting temperature. While the bivorous Brachionus calyciflorus Pallas, 1766, and a Baikal plankton is dominated by herbivorous Epis- green alga, ingestion rates increased for both of the chura baikalensis Sars, 1900, predatory C. kolensis consumers, but the greatest increases occurred at and a variety of herbivorous rotifers can occasionally different temperature ranges; thus, outcomes of their become more numerous (Kozhov, 1963; Pomazkova interactions would differ depending on the range and & Kuzevanova, 1989). Perhaps because the pelagic rates of temperature change (Seifert et al., 2015). waters are frequently a near monoculture of Epischura Plankton community structure and function may (Izmest’eva et al., 2016), the ecology of rotifers in respond to warming most strongly in cold lakes that Baikal, including interactions with their prospective have been historically dominated by coldwater taxa, predators such as Cyclops, is not well documented in such as Lake Baikal in Siberia (Moore et al., 2009). In the international scientific literature. Cyclopoid–ro- addition to being the world’s deepest and most tifer interactions can be highly species-specific due to voluminous lake, Lake Baikal is also the oldest and the many predator avoidance strategies that rotifers most biodiverse lake, and exhibits exceptionally high exhibit as well as variation in recruitment–temperature occurrences of endemism (Kozhov, 1963; Kozhova & relationships among zooplankton species (Brandl, Izmest’eva, 1998). The endemic Baikalian plankton 2005; Zhang et al., 2015). Increasing surface temper- tend to be coldwater stenotherms. They are well atures in Lake Baikal in conjunction with increasing adapted to life under ice for half the year where predatory cyclopoid abundance likewise could inten- average temperatures are about 1°C (e.g., Bondarenko sify cyclopoid predation pressure on rotifers. The et al., 2006; Melnik et al., 2006; Hampton et al., 2008), relative paucity of knowledge on ecology of Baikal’s with optimal growth rates at temperatures below 10°C rotifers and the potential for characteristics of Baikal for prominent endemic plankton species (Kozhov, populations to have diverged from those found 1963; Richardson et al., 2000). However, the highest elsewhere together heighten the need to focus on annual diversity of rotifer assemblages occurs in the interactions for these species under changing temper- late-summer and fall months (Pomazkova & Kuze- atures. We exposed Gastropus stylifer Imhof, 1891 vanova, 1989) when surface temperatures average and Keratella cochlearis Gosse, 1851 to predation by about 8°C and can occasionally exceed 16°C (Hamp- co-occurring cyclopoid copepods (C. kolensis), and ton et al., 2008), and cold-tolerant endemics co-occur compared these interactions with cyclopoid response 123 Hydrobiologia to copepod nauplii. The two central objectives of our different location. These samples for plankton enu- experiments were (1) to measure outcomes of preda- meration and community description data were col- tor–prey interactions for each prey type, and (2) to lected from the long-term plankton monitoring site determine whether these outcomes changed in ‘‘Point No. 1’’ (51°5204800 N, 105°0500200 E) on August response to temperature. We hypothesized that the 19, 2015, where a team of researchers from the Irkutsk less evasive rotifers would be consumed at higher rates State University’s Biological Research Institute has than nauplii by C. kolensis and that the results of sampled plankton every 7–10 days for more than predator–prey interactions may change across a tem- seven decades (Silow et al., 2016). Zooplankton perature gradient as movement rates of predators and samples in this long-term program are collected using prey change in a taxon-specific manner. a 100 lm zooplankton net, which can allow smaller rotifers to pass through the mesh. Samples were collected between 0 m (15.0°C) and 10 m (14.5°C) Methods and immediately preserved with formalin. Samples were then concentrated over a period of 5 days. Our overarching approach was to collect experimental Analysis of the sample consisted of accounting for the animals in the field, starve individual cyclopoid presence and abundance of each species within one- predators, and expose them to rotifer and nauplii prey unit volume for that sample collection. Systematic in both an observational experimental
Details
-
File Typepdf
-
Upload Time-
-
Content LanguagesEnglish
-
Upload UserAnonymous/Not logged-in
-
File Pages10 Page
-
File Size-