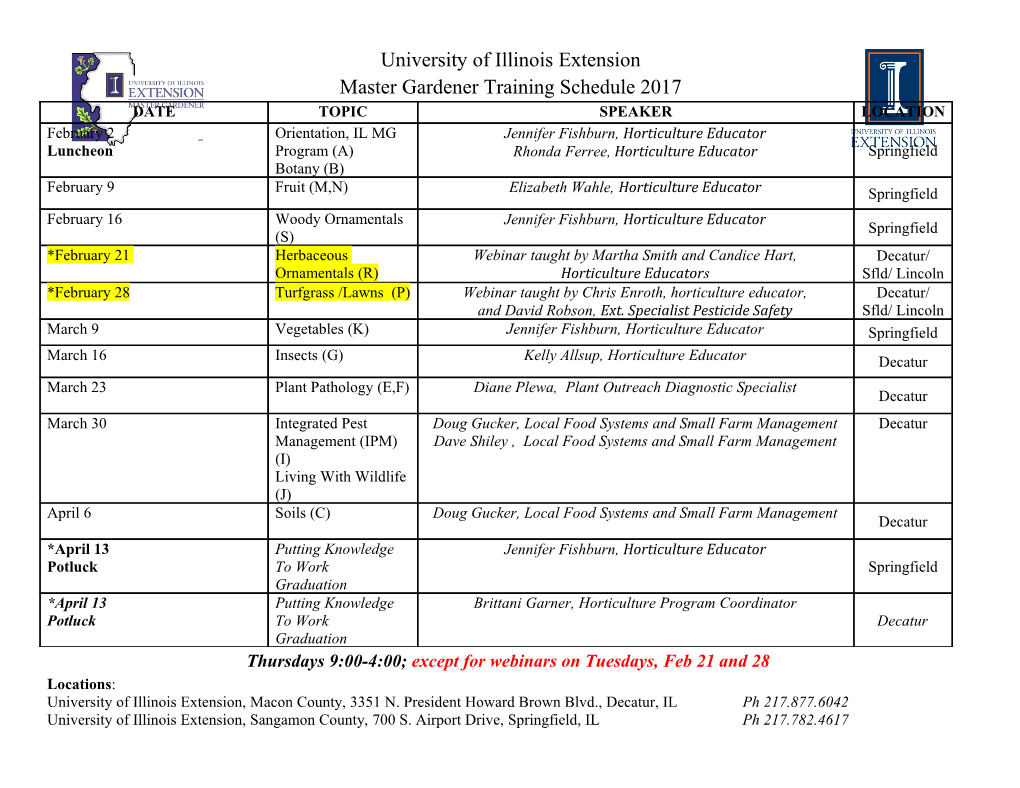
www.nature.com/scientificreports Corrected: Author Correction OPEN Accurate signal-source localization in brain slices by means of high- density microelectrode arrays Received: 27 July 2018 Marie Engelene J. Obien 1,2,3, Andreas Hierlemann 1 & Urs Frey 1,2,3 Accepted: 28 November 2018 Extracellular recordings by means of high-density microelectrode arrays (HD-MEAs) have become Published online: 28 January 2019 a powerful tool to resolve subcellular details of single neurons in active networks grown from dissociated cells. To extend the application of this technology to slice preparations, we developed models describing how extracellular signals, produced by neuronal cells in slices, are detected by microelectrode arrays. The models help to analyze and understand the electrical-potential landscape in an in vitro HD-MEA-recording scenario based on point-current sources. We employed two modeling schemes, (i) a simple analytical approach, based on the method of images (MoI), and (ii) an approach, based on fnite-element methods (FEM). We compared and validated the models with large-scale, high- spatiotemporal-resolution recordings of slice preparations by means of HD-MEAs. We then developed a model-based localization algorithm and compared the performance of MoI and FEM models. Both models provided accurate localization results and a comparable and negligible systematic error, when the point source was in saline, a condition similar to cell-culture experiments. Moreover, the relative random error in the x-y-z-localization amounted only up to 4.3% for z-distances up to 200 μm from the HD-MEA surface. In tissue, the systematic errors of both, MoI and FEM models were signifcantly higher, and a pre-calibration was required. Nevertheless, the FEM values proved to be closer to the tissue experimental results, yielding 5.2 μm systematic mean error, compared to 22.0 μm obtained with MoI. These results suggest that the medium volume or “saline height”, the brain slice thickness and anisotropy, and the location of the reference electrode, which were included in the FEM model, considerably afect the extracellular signal and localization performance, when the signal source is at larger distance to the array. After pre-calibration, the relative random error of the z-localization in tissue was only 3% for z-distances up to 200 μm. We then applied the model and related detailed understanding of extracellular recordings to achieve an electrically-guided navigation of a stimulating micropipette, solely based on the measured HD-MEA signals, and managed to target spontaneously active neurons in an acute brain slice for electroporation. Extracellular recording by means of in vitro high-density microelectrode arrays (HD-MEAs)1–9 has become a powerful tool to resolve subcellular details of single neurons in active networks, grown from dissociated cells, using the spatial information of extracellular action potentials (EAPs). Culturing cells directly on the electrode array allows for detection of neuronal signals along a 2-dimensional plane at high signal-to-noise ratio, so that small axonal signals propagating along axonal arbors can be tracked10–13. In brain-slice microelectrode-array (MEA) experiments, however, the active neurons of interest are inside the tissue slab and feature a 3D arrange- ment, while the viable cells are a few tens of micrometers away from the electrodes14,15. Localization of extra- cellularly recorded active units or neurons in brain tissue is a known challenge, both experimentally and computationally16–18. An accurate localization of the detected cells in brain slices could facilitate the classifcation of the respective cell types and allow for manipulation of target cells by e.g., electroporation, electrical stimula- tion, local pharmacology application, or other interventions. In this work, we study how signal sources in brain slices can be detected by HD-MEAs in order to establish an accurate localization method of the signal sources. We used a known and simple source in all experiments and models—a point-current source. 1Department of Biosystems Science and Engineering, ETH Zurich, Basel, Switzerland. 2RIKEN Quantitative Biology Center, Kobe, Japan. 3MaxWell Biosystems AG, Basel, Switzerland. Correspondence and requests for materials should be addressed to M.E.J.O. (email: [email protected]) SCIENTIFIC REPORTS | (2019) 9:788 | DOI:10.1038/s41598-018-36895-y 1 www.nature.com/scientificreports/ To understand the correlation between brain slice signals and HD-MEA data, we developed models of the HD-MEA recording environment and, importantly, we compared the models with actual high-spatial-resolution experimental data obtained from HD-MEAs. Previous work by Ness et al. presented a modeling-only study, which included slices on traditional MEAs19. Assuming an insulating MEA surface, the method of images (MoI)20–22 was applied to the volume-conductor theory20 in order to analytically compute the electric potentials on the MEA surface. Te MoI model was then compared to fnite-element-method (FEM) simulations. We here extended the proposed MoI and FEM models by considering factors that we could control in our experimental setup, such as the height of the saline bath, the conductivity of the saline, the location of acute cerebellar slice layers with respect to the point source, and the location of the reference electrode. To compare and validate the models, we performed experiments with both, saline only and acute brain slices in culture solution by using HD-MEAs with 11,011 electrodes (3,150 electrodes per mm2)1 for recording and a stimulating glass micropipette as a point-current source. Several factors afect the electrical potential landscape on MEAs in general, whether the signal is coming from a simple point source or from a complex source, such as a neuron. For cell-culture experiments, the amplitude of neuronal signals was observed to increase when mineral oil—a non-conductive fuid normally used to prevent evaporation of culture medium—was poured onto the MEA dish (unpublished data). Similarly, it has been shown that a sheet of glia can serve as an insulator layer and increase the spike amplitude of cultured cells23. Microtunnels made of poly(dimethylsiloxane) or PDMS also amplify action potentials propagating along axons24–26. In this study, we analyzed how diferent saline heights, with the saline-to-air interface acting as an insulator, afect the amplitude of MEA signals. For acute brain slice experiments, the reduced conductivity of brain tissue with respect to saline has to be considered and substantially afects the amplitude of the recorded signals. Brain tissue has been mostly considered and modeled as homogeneous and resistive material21,27,28. A thorough understanding of the HD-MEA extracellular environment is a requirement for accurate localiza- tion of signal sources in brain slices. In detail, we studied if a realistic HD-MEA model could improve the accu- racy (see defnition in Methods) of localizing the micropipette in saline and in an acute brain slice. We separated the localization problem into two parts: (a) x-y localization and (b) z localization. We used 2D Gaussian ftting for the x-y localization, assuming the brain slice being homogeneous in the x-y direction, and MoI and FEM models to assess the z-distance (distance between the source and the HD-MEA surface) of the point source in saline and in the presence of an acute brain slice. We ftted the measured potential distribution to the simulated potential distributions of both MoI and FEM models to estimate the z-distances. To demonstrate an application and the performance of the localization algorithm, we targeted single sponta- neously active neurons in acute cerebellar slices for electroporation with a micropipette using a micromanipula- tor. Tis experiment served as a proof of concept for solely using the signals of HD-MEAs to steer micropipettes with high accuracy and to demonstrate that an all-electrical electrophysiology and micropipette control system could be realized. Instead of using a microscope to visualize the cells and the micropipette tip, the HD-MEA acted as an electrical-imaging camera to localize both, the electrically active cells and the micropipette. Te combined micropipette-HD-MEA approach ofers prospects of an electrically guided automated patch-clamp29, in contrast to blind30–32 and visually33 guided approaches. Future applications of this method aim towards high-throughput single-cell manipulation and analysis. Results We developed a localization method that allows for accurate identifcation of the 3D position of a micropipette, representing a point source, above the HD-MEA surface in the presence of saline or acute brain slices. For sim- plicity, we separated the localization problem into two parts: x-y localization and z localization. In this way, we were able to extract the x-y location of the point source independently of its z location. Estimating the x-y location is straightforward, based on the peak potential, and we will show that accurate localization can be achieved by Gaussian ftting. For z localization, we performed the following steps: First, we acquired a large experimental HD-MEA data set by recording the signal characteristics and potential landscape of a stimulating micropipette located at various defned z-distances in saline above the microelectrode array. Te obtained data were then used to derive an experimentally determined point spread function (PSF), and served as a basis for fnding the best HD-MEA model for use with the localization algorithm. Second, we modeled the recorded HD-MEA signals by using two methods19, (1) analytical equations based on volume-conductor theory and the method of images (MoI), and (2) a fnite-element method (FEM). Using these two methods, we explored diferent parameters that can afect the HD-MEA signals. Tird, we determined parameters in the MoI and FEM models that best described our experimental conditions, such as saline column height, tissue thickness and ground location.
Details
-
File Typepdf
-
Upload Time-
-
Content LanguagesEnglish
-
Upload UserAnonymous/Not logged-in
-
File Pages19 Page
-
File Size-